Surface-enhanced Raman spectroscopy is the emerging star of Raman spectroscopy, promising the ultimate in sensitivity, early disease diagnosis and more.
After years of exclusion as an academic technique, Raman spectroscopy has finally, firmly taken its place as an important analytical tool used for applications ranging from noninvasive imaging for diagnostics to in-field sensing for the detection of drugs and explosives. Today’s Raman spectroscopy market offers systems ranging from easy-to-use handheld systems dedicated to one application to automated analytical systems or multipurpose high-end research systems.
Although advances in CCD detectors, filters and inexpensive and easy-to-use diode lasers available over a wide wavelength range have advanced Raman spectroscopy, the technique has certain limitations: It is not considered very efficient in terms of sensitivity and acquisition times. Indeed, compared with a fluorescence signal, for example, Raman does not look very impressive, even if some good Raman scatterers can give a signal in a few milliseconds or less.

Example of SERS in action: Bottom shows a Raman spectrum of dithiothreitol (DTT) in aqueous solution at 5-mM concentration – such concentration is too low for standard (nonenhanced) Raman spectroscopy to provide any meaningful chemical information. Middle shows the Raman spectrum of solid (powdered) DTT. The top shows the SERS enhanced spectrum of 5-mM DTT, clearly revealing detection at this low concentration. Courtesy of ©2013 Horiba Scientific.
But one technique is currently causing a buzz at spectroscopy conferences and dominating scientific reviews: surface-enhanced Raman spectroscopy (SERS). Heralded as one of the answers to low Raman signals, SERS was discovered in the 1970s, but it wasn’t until single-molecule detection was demonstrated in the 1990s that interest really took off.
“Single-molecule detection with SERS has been a turning point in the history of SERS. It was obviously enabled by huge developments in technology in terms of laser, optics and confocal microscopy – but since then, SERS hasn’t looked back,” said Dr. Sumeet Mahajan, senior lecturer at the Institute of Life Sciences and Department of Chemistry at the University of Southampton in England. “Single-molecule detection with SERS is significant, as it is the ultimate in sensitivity.”
The potential of single-molecule detection means that very low levels of the target molecule can be discovered, which makes very early stage detection of disease possible, and today, many believe that SERS is emerging as the most promising technique for developing Raman-based sensors.
“SERS is really the cutting edge of Raman spectroscopy,” said professor Katrin Kneipp of Technical University of Denmark. “Usually, Raman spectroscopy was a method for structural characterization of matter, requiring relatively large amounts of matter. SERS turns it into a single-molecule tool.”
Applications of SERS
Although diagnostics is the big target, any application in which the ability to capture and analyze a small particle or moiety would work, such as contamination in water, drugs/explosives detection in homeland security, chemical/biological weapons and agents, or even art preservation.
Last year, at Imperial College London, Dr. Joshua B. Edel’s group, in collaboration with professor Alexei Kornyshev, showed that SERS could detect one analyte molecule within the local surrounding of 1016 water molecules.1
The collaborators also recently developed technology that opens the door to devices that are compact, reusable and easy to assemble, and that could have a range of uses including detecting illegal drugs, explosives, pollutants in rivers or nerve gases released into the air.
“For example, our platform could detect tiny traces of explosives or other illegal substances left behind by criminals on the surfaces they touch,” said Edel, who is senior lecturer in micro- and nanotechnology. “The advances made by this team would help law enforcers to identify and deal with such activities involving illegal substances.”

(Left) A schematic of SERS from nanoparticles inside cells. The SERS spectra can be used to generate a false-color ‘chemical map’ by plotting the distribution of various peaks across the sample area. In this case, the biomolecular distribution of different protein and oligonucleotide vibrations is shown inside a cell. (right) A normal optical image of the cell corresponding to the adjacent SERS map (center). Courtesy of Anna Huefner, University of Cambridge, and Dr. Sumeet Mahajan, University of Southampton.
The ideal substrate
Even though the potential reach of SERS is undoubtedly great, much of the current research focuses not on applications but on providing a SERS active-surface chemistry that not only enhances the Raman signal, but also targets and captures specific molecules.
If this were successful, it could enable routine diagnostics for disease states; e.g., capturing and enhancing the signal for marker proteins.
“There are many variants on a SERS substrate. The majority are based on solid-state top-down approaches and involve the fabrication of a periodic array of nano-objects made from a SERS active surface such as silver or gold,” Edel said. “Other strategies involve bottom-down approaches such as the self-assembly of nanoparticles either on a solid substrate or at a liquid-liquid or even liquid-air interface.”

The self-assembly of nanoparticles at liquid-liquid interfaces has recently emerged as a promising platform for tunable optical devices, sensors and catalysis. Courtesy of Benjamin Miles.
An ideal substrate can be produced in numerous ways, but the aim is the same: to produce reproducible and reliable structure in the substrate so that it gives repeatable results. A SERS signal is affected by so many parameters, including size and shape of the particles, laser wavelength, spot size, binding chemistry and the number of molecules actually being probed. This means that a small change anywhere can produce large variations and make quantification difficult.
“The ideal SERS substrate has homogeneous enhancement across the surface which can be quantified. This is key in defining a limit of detection. Any sensor out there needs to provide a reliable pass/fail signal, which means the threshold needs to be clear and consistent,” said Renata Lewandowska, Raman applications manager at Horiba Scientific, a spectroscopic instrument company based in Kyoto, Japan. “The issue today is that SERS enhancement is so huge, it is difficult to compare bulk and SERS signals. Moreover, SERS is used often in the situation where ‘classical’ Raman does not give any signal; thus, direct comparison is often not possible.”
Nanoparticles enhance SERS signal
The latest focus for SERS scientists is the investigation of nanoparticles of different shapes and sizes to allow for fine-tuning of plasmonic properties, and to increase the enhancement levels within “hot spots” in the substrate.
Really high SERS enhancement requires small aggregates formed by at least two nanoparticles, Kneipp said. Then, the gap size between the particles appears a key parameter for achieving an extremely high enhancement level.
Particle shapes (such as rods, cubes, spheres, prisms and tetrapods, etc.) have all been shown to exhibit unique properties and can provide high enhancement level at points of high curvature, such as the corners of a prism or the end of a tapered rod forming a “tip.” Researchers in China have published information on SHINERS (shell-isolated nanoparticle-enhanced Raman spectroscopy) that can make SERS universally applicable to surfaces with any composition and any morphology.2
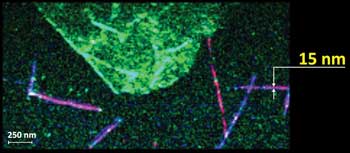
This TERS hyperspectral image shows 15-nm spatial resolution on carbon nanotubes. Courtesy of ©2013 Horiba Scientific and AIST-NT.
Professor Zhong Qun Tian of Xiamen University in China and colleagues have developed a technique to fabricate SHINERS nanoparticles that could address some of the problems associated with traditional SERS substrates. It could even provide a more robust medium for the analysis of pesticide residues and applications in the materials and life sciences as well as for the inspection of food safety, drugs, explosives and environmental pollutants.
What’s next: TERS
Arguably the most significant development since the demonstration of singe-molecule detection is tip-enhanced Raman spectroscopy (TERS), in which a metal tip enhances the molecules’ Raman signals by moving the tip closer to the area under investigation.
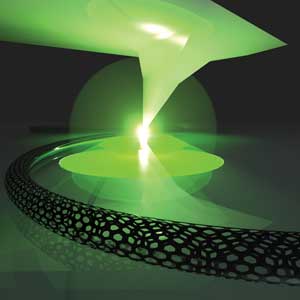
In tip-enhanced Raman spectroscopy (TERS), a metal tip enhances the molecules’ Raman signals by moving the tip closer to the area under investigation. Courtesy of ©2013 Horiba Scientific.
In this setup, the molecules themselves do not need to be on the tip but can be on a dielectric or metal surface, which means that SERS no longer is confined to studying interfaces made of coinage metals only and even allows study of non-nanostructured surfaces (flat, single crystalline) as well as natural (dielectric) surfaces such as membranes of cells and the like.
“Whilst still a relatively high-end technique, it does have great potential as a label-free chemical imaging technique for the nanoscale, for looking at the next generation of nanomaterials (such as nanotubes, graphene, nanowires) and, in the field of biology, for looking at cell membranes, fibrils and down to DNA, RNA, etc.,” said Emmanuel Leroy, Raman-AFM and TERS product manager at Horiba Scientific.
There is no doubt that SERS is headed for continued growth for many years to come, and that further developments of the technique or its delivery will propel SERS forward. The issue of deep-tissue penetration, which involves recording SERS from deep within, is being solved by innovations such as spatially offset recordings (called SE-SORS).
Remote monitoring and telemetry with SERS, combined with optical fibers, is a real possibility for routine use, and SERS, using unconventional materials such as metal oxides and high-refractive-index materials as substrates, has the potential to enlarge the landscape of applications to catalysis (including photo- and electrocatalysis). This has been explored in a paper by Mahajan and colleagues.3
Biomedicine offers innumerable questions, many of which can potentially be answered by playing to the strengths of SERS, Mahajan said. A case in point is his team’s demonstration of SERS inside the nucleus of neurons, using functionalized nanoparticles detecting subtle biochemical changes for distinguishing morphologically similar and closely related cell types, not easily possible without complicated techniques.4
“I believe that the applications of SERS in biology and medicine will be the key drivers of its growth over this decade,” he said. “Diagnostics, both in vitro/ex vivo, as well as in vivo is another big growth area. SERS is already beginning to make an impact in the former, and this is only going to increase as biomedical scientists and clinicians become increasingly aware of the capabilities of SERS.”
References
1. M.P. Cecchini at al (2013). Self-assembled nanoparticle arrays for multiphase trace analyte detection. Nat Mat, Vol. 12, pp. 165-171 (doi: 10.1038/nmat3488).
2. J.F. Li (2013). Surface analysis using shell-isolated nanoparticle-enhanced Raman spectroscopy. Nat Pro, Vol. 8, pp. 52-65 (doi: 10.1038/nprot.2012.141).
3. L. Li et al (2012). Metal oxide nanoparticle mediated enhanced Raman scattering and its use in direct monitoring of interfacial chemical reactions. Nano Lett, Vol. 12, Issue 8, pp. 4242-4246 (doi: 10.1021/nl302029p).
4. A. Huefner et al (2013). Intracellular SERS nanoprobes for distinction of different neuronal cell types. Nano Lett 2013, Vol. 13, Issue 6, pp. 2463-2470 (doi: 10.1021/nl400448n).
Top applications of SERS
• DNA analysis, mutation detection and genotyping.
• Biomolecular identification: detection of glucose, amino acids, peptides and many other such molecules.
• Environmental applications such as detection of organic pollutants and monitoring water quality.
• Pharmaceuticals: quality control and counterfeit detection.
• Life sciences: cell- and tissue-based analysis.
• Forensics: detection of anthrax spores.
• Security: drugs/explosives/chemical or biological weapons detection.
• Art preservation and conservation: in situ identification of trace-level dyes and pigments without damage.