Planar 4G optics offer increased control over broadband light with micrometer-thin, clear, and haze-free films switchable between different optical states.
NELSON TABIRYAN, BEAM ENGINEERING FOR ADVANCED MEASUREMENTS CO.
A micrometer-thin film coated over a flat glass substrate can deflect a light beam in the same way as a prism. This film can be fabricated to be structurally smooth, at a molecular level, and as clear and haze-free as a
polished window of fused silica or
sapphire.
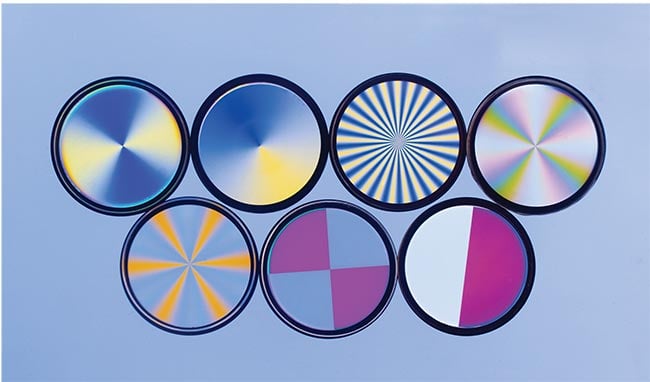
Courtesy of BEAM Engineering for Advanced Measurements Co.
With an even wider transmission spectrum than classic optical windows, these flat prisms and lenses — as well as optical vortices, axicons, patterned phase plates, beam shapers, and numerous custom optics — find use for a variety of demanding applications. Many of these applications involve high-energy laser beams and take place in harsh environmental conditions, including in space and on the battlefield.
The components and devices that comprise this class of optics underscore a revolution in basic optical technology. Since the turn of the century, this has been marked by the feasibility to achieve control over broadband light over a large range of angles without absorption and scatter losses, to be switchable between different optical states independent of light polarization, and to achieve large aperture.
Diffraction efficiency exceeding 99% throughout the visible and IR bands for a wide range of angles, obtained in films of thickness(es) comparable to the wavelength of light, indeed seemingly defies textbook properties of conventional optics. However, this combination is inherent to diffractive waveplates — optical films that feature spatially varied orientation(s) of an optical anisotropy axis1,2. The phase of a circularly polarized light beam propagating through a waveplate gets modulated following the optical axis alignment pattern for wavelengths meeting the half-wave retardation condition.
As a result, the molecular alignment patterns that can be revealed between polarizers determine the optical function of the diffractive waveplate (Figure 1).
Figure 1. Alignment patterns of an optical anisotropy axis in a diffractive waveplate lens (a) and in an axicon (b). The components appear as transparent windows (c) unless viewed through polarizers (d). Courtesy of BEAM Engineering for Advanced Measurements Co.
4G: the ultimate planar optics
The proliferation of planar optics is a result of three previous generations of optics, which themselves can be viewed iteratively: shape modulation (first generation); the modulation of refractive indices, such as in gradient index lenses (second generation); and the modulation of effective birefringence, such as in liquid crystal displays (LCDs, third
generation). Though pioneered to overcome the low-light efficiency of LCDs caused by absorption by polarizers, the diffractive waveplates that mark the fourth generation of optics now find use in AR/VR glasses, adaptive ophthalmic glasses, and lidars. Applications span defense/aerospace, manufacturing, and remote sensing3.
As noted by Bernard Kress, director for XR engineering at Google and 2023 SPIE president, there is no “Moore’s law in optics.” In other words, unlike in the realm of semiconductor devices, nature itself has not provided other opportunities to control light (excluding gravitation).
Liquid crystals are best suited for the fabrication of 4G optical components. First, their high elasticity — liquid crystals do not “break” — enables the production of modulated structures of optical anisotropy axis with micrometer resolution(s) using fast processes and inexpensive materials. Second, the optical anisotropy — the difference in refractive indices for light that is polarized parallel and perpendicular to the optical axis of liquid crystals — is as large or even larger than the change of refractive index during water evaporation. Thus, micrometer-thin liquid crystal layers can introduce half-wave retardation into a light beam for different parts of the spectrum.
Also, liquid crystal materials are highly transparent from UV to MIR wavelengths. Transmission lines even occur at LWIR and terahertz bands, and the same materials can be used to produce optics at different bands. This means that only a fraction of a milligram of a liquid crystal material per 1 sq cm of aperture is required to produce half-wave retardation films and coatings, supporting prisms and lenses of high efficiency and optical power. The spatial modulation of molecular alignment is commonly produced using UV-visible blue light, with exposure energy as small as 1 mJ/cm2 obtained even in a single laser pulse or under sunlight.
The advantages of using liquid crystals in the fabrication of 4G optics extend to liquid crystal polymer films. These films can be released and transferred to different substrates or framed into pellicle optics; the substrates used in production are fully recyclable.
Custom optics and same-day fabrication
Retardation, alignment, and/or the number of films that can be integrated into diffractive waveplates enables a wide customization range of their diffraction efficiency. This provides an opportunity to control the propagation of light beams within desired wavebands without affecting the propagation of light at wavelengths outside of these bands. Spectrally selective lenses fabricated in this way are combined in a single film to focus light at the RGB wavelengths into the same focal plane, as shown in Figure 2, which also shows an image of a white light LED array obtained via a polychromatic 4G lens.
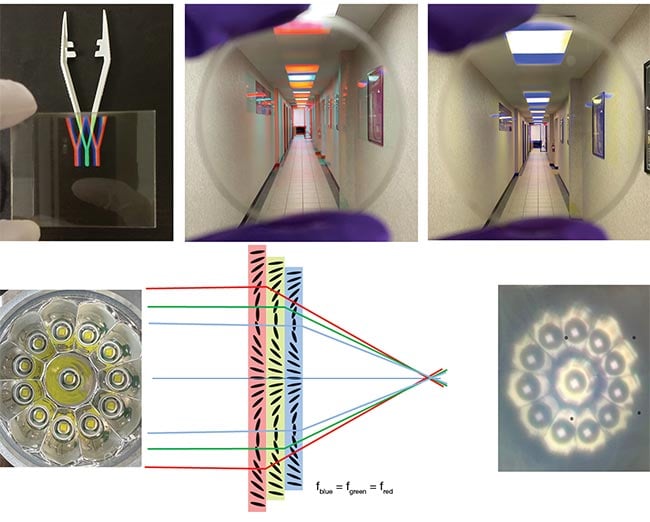
Figure 2. Functionality of spectrally selective cycloidal diffractive waveplates — planar analogs of a prism deflecting specific spectral bands only (top row). The structure of a multispectral lens comprising planar lenses optimized for diffraction of different spectral components of incident white light is schematically shown, along with the pattern obtained in the focus of such a lens illuminated by a flashlight with circular distribution of LEDs (bottom row). Courtesy of BEAM Engineering for Advanced Measurements Co.
The spectra in Figure 3 represent diffractive waveplates that are optimized for UV and visible broadband optics for circularly polarized light, and broadband IR optics for unpolarized light. Basic diffractive waveplates diffract unpolarized or linearly polarized light into two beams of orthogonal circular polarization. This feature is used, for example, for instantaneous polarimetry and circular dichroism spectrometry. The architecture of diffractive waveplates, the slant angle of planes of equal molecular alignment, and the birefringence of the material can be chosen to achieve Bragg condition for one of the diffracted beams, as well as “anti-Bragg” condition, which cancels the diffraction for the second beam. Such films may then be combined for polarization-independent deflection or lensing with no compromise to diffraction efficiency.
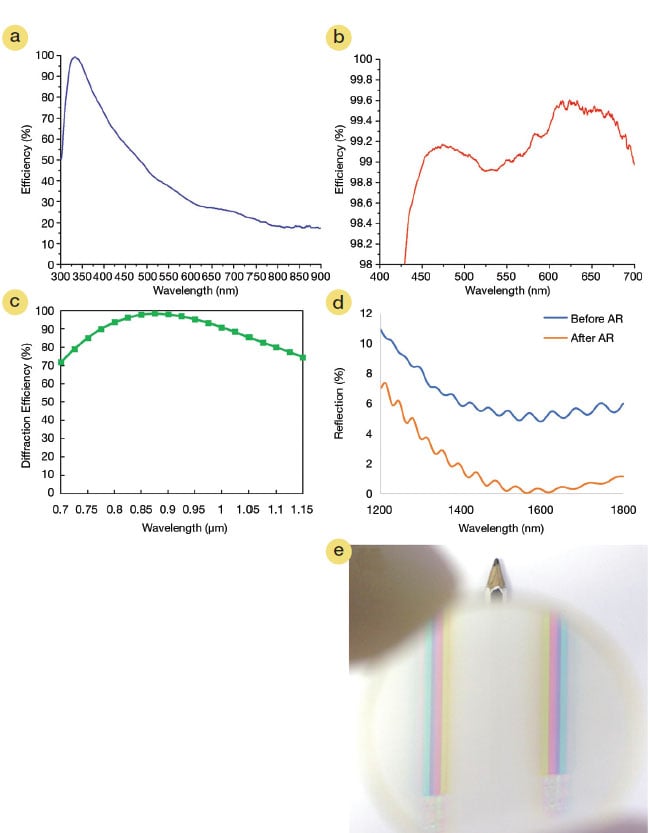
Figure 3. Spectral characterization of diffractive waveplates. A diffractive waveplate optimized for a UV wavelength (a); efficiency of a visible broadband diffractive waveplate (note the 98% to 100% scale) (b); efficiency for unpolarized light (previous spectra relate to circular polarization) (c); a liquid crystal polymer waveplate with antireflective (AR) coating (d); a performance demonstration of a visible broadband cycloidal diffractive waveplate by looking through the optic onto a black and white pencil (e). Courtesy of BEAM Engineering for Advanced Measurements Co.
Figure 3 also demonstrates near-100% throughput of a diffractive waveplate
with an antireflection (AR) coating that can be deposited on liquid crystal polymers. The continuous and smooth surface of these polymers, as well as the very high levels of mechanical strength and thermal and chemical resistivity of liquid crystal polymers, enables this deposition. Such broad spectral bandwidth translates in turn into a wide angular range of high
efficiency: The image of a clock on the wall illuminated by white ambient light is not leaking through a broadband 4G prism even at extreme/steep angles (Figure 4). Due to a high diffraction efficiency, the sun can be observed through a cycloidal diffractive waveplate with the naked eye, without dark filter glasses (Figure 5).
The simple relationship between the phase and the alignment pattern of the optical anisotropy axis means that the physical modeling of 4G optics lasts only minutes and can be presented without complex formulas. Simply, phase equals twice the angle of orientation.
This phase, known since the 1950s, is referred to as geometrical phase (and/or Pancharatnam phase, and/or Pancharatnam-Berry phase). However, spatial modulation, and not phase itself, influences light propagation. This notion was not perceived in the early years of geometrical phase research. It is possible that the alternative names of diffractive
waveplates may have hindered the development of the technology, veiling its
relative simplicity with complex definitions and descriptions.
No matter the case, 4G optical components of desired functionality can be modeled, designed, and produced within minutes, even in a large area. This fabrication technology today retains many commonalities with established LCD fabrication technology. This is particularly evident for liquid crystal-based switchable 4G optics, such as lenses of switchable focal length, switchable beam shapers, and prisms of switchable deflection angle.
Additionally, the maturity of the LCD industry ensures that liquid crystal physics and chemistry are well understood, and that materials optimized for all different electro-optical properties are available, and most often at low cost(s). Even specialized liquid crystals are
available to be purchased for roughly $100/mL, a quantity sufficient to produce a lens of 1000 sq cm.
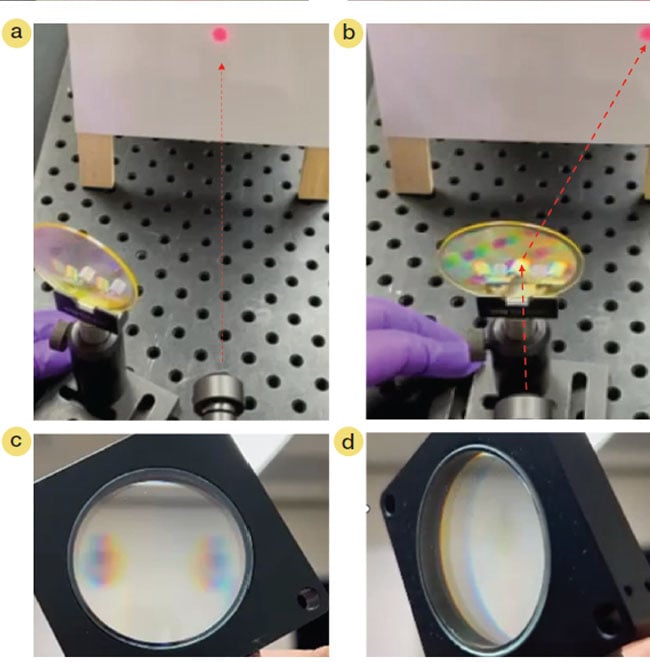
Figure 4. The cycloidal diffractive waveplate coated over a glass substrate of a 2-in. diameter. It is out of the beam (a) and brought into the beam (b), deflecting it as a prism. High efficiency at very large angles is demonstrated (c, d) by looking at a diffraction pattern of a clock on the wall at large angles. No leakage is observed. Courtesy of BEAM Engineering for Advanced Measurements Co.
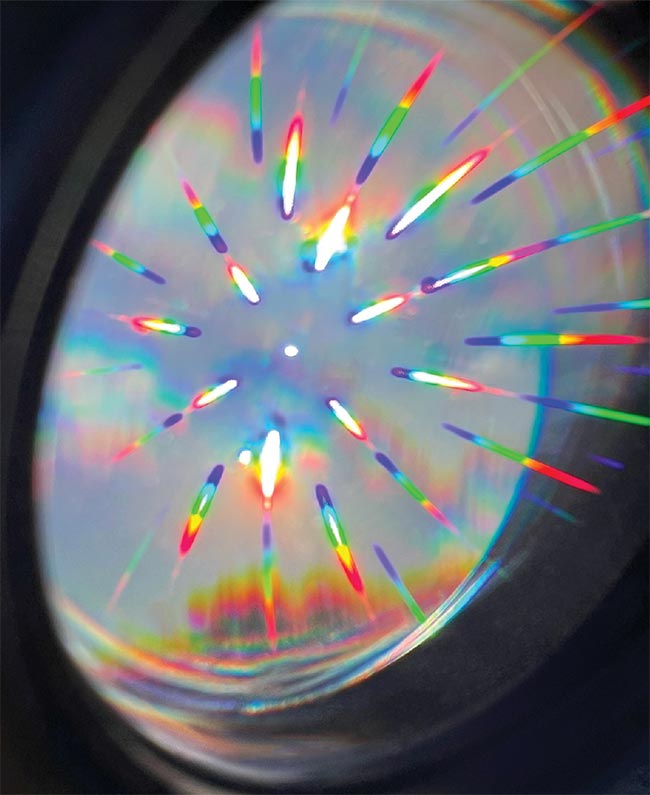
Figure 5. Imaging the sun through a pair of diffractive waveplates. Courtesy of BEAM Engineering for Advanced Measurements Co.
Critical fabrication technologies including spin and inkjet coaters, polarization holography systems, and spatial light modulators, as well as the more specialized spatial light polarization modulators, are also widely available at affordable costs. Due to the use of polarization holography, some of those components are also referred to as polarization gratings, though not all polarization gratings are diffractive waveplates.
Ultimately, large-scale production is feasible without reliance on sensitive holographic recording systems. The use of polarization lithography — printing through polarization converter masks with low-power incoherent light sources — is an established method for fabrication at scale.
Reflective geometrical phase optics
Liquid crystals also enable geometrical phase modulation upon reflection, opening additional opportunities to deploy these optics4. This quality is obtained when circularly polarized light is
reflected from a helical bandgap formed by a chiral liquid crystal, of which the periodic structure provides Bragg reflection. At the same time, the helical nature of the molecular alignment pattern makes the bandgap sensitive to the handedness of the circular polarization of the light; namely, only light that is circularly polarized according to the handedness of the helix is reflected when its wavelength is within the bandgap.
Light of orthogonal circular polarization propagates through the material without being affected, as does light of non-bandgap wavelengths. In this way, the chiral liquid crystals function as
perfect reflective circular polarizers.
Further, since the rotation of the helix around its axis changes the phase of a circularly polarized incident light upon reflection, spatial modulation of the helix alignment may focus, deflect, or otherwise manipulate light upon reflection. As with transmissive optics, polarization-independent performance is achieved by combining chiral liquid crystals of right- and left-handedness, and spectrally broadband performance is achieved by combining chiral liquid crystals of different Bragg wavelengths or by chirping the helix. From a manufacturing standpoint, and ultimately for performance of the optic, avoiding the need to maintain
half-wave retardation condition(s) results in a significant advantage.
Chiral liquid crystals are Bragg gratings of 0.1 and higher effective refractive
index modulation versus 0.001 and
lower for conventional (glass) volume Bragg gratings. This has important consequences. First, high contrast reflection is obtained in a thin film of several micrometers, compared with millimeters for conventional Bragg gratings. Second, the width of the bandgap can be as wide as ~10 to 50 nm for visible wavelengths. Third, the bandgap does not possess high angular selectivity of conventional volume Bragg gratings. Moreover, both the Bragg wavelength and the bandgap width in chiral liquid crystals are easily controlled by material engineering or external conditions.
4G vs. metaoptics
Typically, in discontinuous structures, such as Fresnel lenses and metalenses
(in this case, referring to all metasurface optics technologies), the non-diffracted light is present in the image in the form
of haze, which restricts the optical
performance of these components. The portion of light that remains unfocused
is a core consideration in optical imaging, and, more specifically, for the function
of the optics used in imaging applications.
Structural discontinuities set fundamental limitations to metalens efficiency. And similarly, functional limitations of metalens technology encompass different considerations, each of which are apt to influence performance5. These factors include the inability to provide near-diffraction-limited performance at a varied range of Fresnel numbers as well as the inability to deliver large focusing power and broadband response in a simultaneous manner6.
Manufacturing metalenses remains a bottleneck too, due to the inevitable presence of defects in their structure comprising billions of pillars of all different shapes and sizes. This is the case even for IR wavelengths. In contrast, 4G optics are manufactured for blue or even UV wavelengths by simply controlling the thickness of the liquid crystal layer.
Metalens manufacturing challenges are also present in the modeling and simulation stages, both of which require considerable computational resources.
Further issues related to metalens manufacturability tie in cost considerations. Development of a so-called
master metalens that could in theory be used for inexpensive replication is
hindered by the need to maintain the sharpness of the features of the nano-micropillars during the pattern transfer processes from the resist to silicon. For example, an efficiency decrease from 45% (master lens) to 6% (for the nanoimprinted replica) has been reported7.
Finally, there is the consideration of time. The fabrication of metasurfaces typically involves several time-consuming processing steps, and potentially the use of hazardous materials.
The holy grail of optics
The holy grail of optics — an optic as clear and efficient as glass shaped into lenses and prisms, yet not as thick and heavy, and overcoming efficiency and bandwidth limitations of diffractive optics and metasurfaces — is now within sight. The spread of this technology is evident in R&D, academic, and industrial settings, and members of all three communities are currently actively involved in the development of 4G optics and optical components.
This development is fueled by the
novel opportunities that feed directly
into the immediate needs of many
modern technologies, enabling large and thin AR glasses that are switchable between multiple focal lengths; electronically switchable ophthalmic lenses; nonmechanical lidars for autonomous navigation; ultralight and compact beam directors for high-energy lasers; and photonic chips used to dynamically process an input light into a light of desired propagation and polarization properties. NASA, DARPA, and other advanced technology organizations are developing and supporting the development of appli-
cations that range from diffractive sails and coronagraph masks for exoplanet imaging to the aerial network of wireless energy transfer.
These achievements, plus a high
level of technology maturity, are largely
a result of collaboration between industry, high-technology organizations, military research, and R&D agencies. The support and active involvement of NASA, the U.S. Army, DARPA and, particularly, the Air Force Research Laboratory, have made it possible to establish the key functional material basis of the technology and to perfect fabrication processes to meet strict production tolerances typical to waveplates and the optical quality demanded by modern applications.
Meet the author
Nelson Tabiryan is a pioneer in liquid crystal photonics developments, an Optica Fellow, and CEO and president of BEAM Engineering for Advanced Measurements Co., founded in 1996; email: [email protected].
References
1. N. Tabiryan et al. (2017). New 4G optics technology extends limits to the extremes. Photonics Spectra, Vol. 51, No. 3, pp. 46-50.
2. N. Tabiryan et al. (2021). Advances in
transparent planar optics: enabling large
aperture, ultrathin lenses. Adv Optical Mater, Vol. 9, No. 5.
3. N. Tabiryan et al. (2019). Diffractive waveplates: introduction. JOSA B, Vol. 36,
No. 5, pp. DW1-DW2.
4. R. Barboza et al. (2016). Berry phase of
light under Bragg reflection by chiral liquid-crystal media. Phys Rev Lett,
Vol. 117, No. 5-29.
5. J.-S. Park et al. (2024). All-glass 100 mm diameter visible metalens for imaging the cosmos. ACS Nano, Vol. 18, No. 4, pp. 3187-3198.
6. J. Engelberg and U. Levy. (June 2021).
Achromatic flat lens performance limits. Optica, Vol. 8, No. 6, pp. 834-845.
7. A.M. Baracu et al. (2021). Silicon metalens fabrication from electron beam to UV-
nanoimprint lithography. Nanomaterials, Vol. 11, No. 9, p. 2329.