Cytometry is quickly evolving by leveraging more laser wavelengths, fluorescent probes, and analytical techniques.
FAROOQ AHMED, CONTRIBUTING EDITOR
The COVID-19 pandemic has put the field of immunology under an international spotlight. Researchers in the field have been rigorously studying our immune system’s response to the SARS-CoV-2 virus to understand how we combat infection. Advancements in immunological detection have relied on one instrument in particular: the flow cytometer.
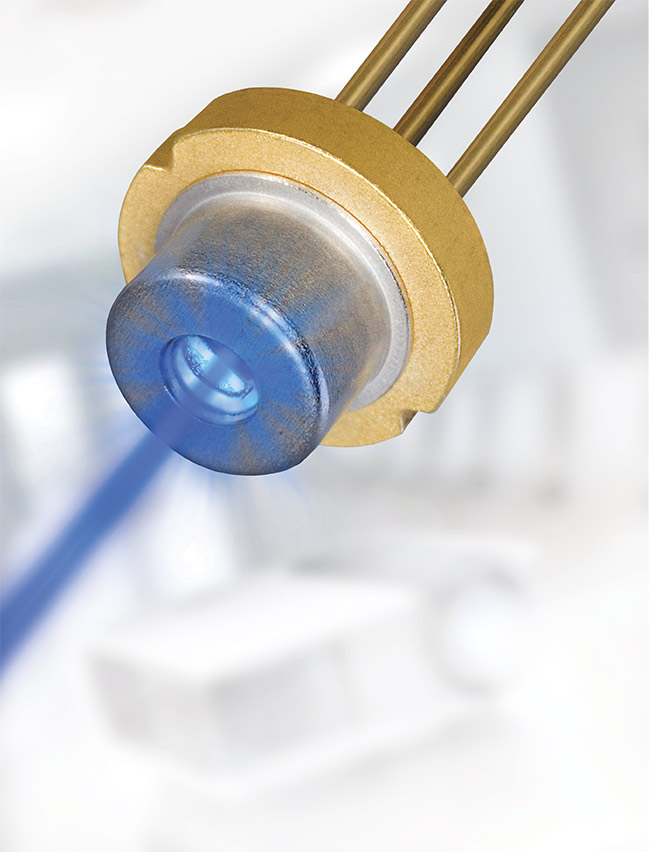
Blue lasers operating at 488 nm, similar to OSRAM’s PLT5 laser diode, contributed to recent research using nanoscale flow cytometry that explores variability in activity of HIV protease in patients infected with the virus. Courtesy of OSRAM Opto Semiconductors.
“Flow cytometry is important to our understanding of all infectious diseases,” said William Telford, who heads one of the National Cancer Institute’s flow cytometry core facilities. “It’s being used to characterize the immune changes that occur in SARS-CoV-2, as well as other viruses.”
Telford said immune cells look remarkably similar under the microscope. Only the proteins they express on their surfaces reveal their subsets: T-cells versus B-cells, activate versus inactivate, disease-specific versus nonspecific, and so on. “And, we’re discovering more and more immune cell markers,” he said.
Giacomo Vacca, president and founder of Kinetic River, echoed Telford’s sentiments on the impact of COVID-19 and immunology. “Especially in fields like immunology, we see applications driving the need for better tools,” Vacca said.
Like most biomedical tools, flow cytometers are complex instruments that rely on the convergence of several scientific disciplines, including fluid mechanics, computer science, and optics and photonics. In a typical application, a sample, such as a heterogenous population of cells, is tagged by fluorescently labeled antibodies and injected into a microfluidic chamber where it passes through a laser beam that excites the fluorophores. Detectors record the emitted and scattered light and send the results to a computer for analysis.
While the technique dates to the 1950s, the devices in current use are nearly unrecognizable from their origins: Some of the most advanced instruments contain up to 10 lasers that emit beams in as many wavelengths.
“We’ve seen fundamental changes to the technology that are going to continue to really affect the field,” Telford said. He has been using the technique as an
investigator and expert for more than three decades, and his facility supports
many of the academic and clinical researchers who work with the National Cancer Institute.
Limitless labels
Over the past decade or so, Telford has witnessed a doubling in the number of cell surface markers and fluorescent probes that researchers are using to define cell populations. “We’re up to 40-color, fluorescent parameter labeling with commercial instrumentation,” he said. “We’re taking advantage of the wide variety of visible laser wavelengths available to us.”
The number of parameters is important, Vacca said, because they allow scientists to finely characterize seemingly similar groups of cells.
This increase in the number of labels, however, poses potential challenges. Fluorophores have long spectral tails that cause their emissions to spill over from one detection channel into another. They consequently need appropriate lasers, detectors, and computational algorithms that can discern between these overlapping signals. Vacca added, “Compensation problems become more compounded the more labels you add.”
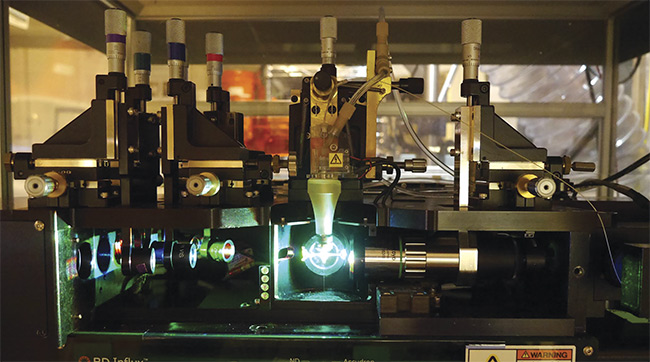
Like most biomedical tools, flow cytometers are complex instruments that rely on the convergence of several scientific disciplines, including fluid mechanics, computer science, and optics and photonics. Courtesy of William Telford.
Flow cytometrists and instrument manufacturers have devised ways to increase the number of labels available to researchers without having to rely on mathematical processes such as color compensation, which is used to distinguish spectral overlap. One method, Telford said, is to push labeling beyond the visible range and into the UV. Vacca agreed: “After solid-state 355-nm lasers were commercially introduced in the late 1990s, flow cytometers offering that excitation wavelength slowly started proliferating.” He added that chemists have developed fluorophores accordingly.
Telford’s lab and others are using spatially separated, continuous-wave lasers to excite fluorophores in the visible and UV regions. He said detector technology is also important, and that his group has started using arrays of avalanche photodiodes to capture fluorescent signals instead of more traditionally used photomultiplier tubes.
Another development that has been gaining traction, Telford said, is spectral flow cytometry. This iteration of the technique uses CCDs for detection, which capture the full spectrum of each excited fluorophore rather than a specific emission wavelength. This allows for finer discrimination between labels. “I think that in 10 years, spectral will be the dominant technology,” he said.
At Kinetic River, Vacca has taken a different approach. To compensate for spectral overlap, his company builds flow cytometers that account for the fluorescence duration or lifetime of each fluorophore — not just its emission wavelength. To do so, however, they had to switch from continuous-wave lasers to a pulsed laser setup.
“Fluorescence lifetime gives us an additional parameter to distinguish molecules with similar spectra,” Vacca said. “You can label cells with multiple fluorophores, all of which emit in the blue region, for example, and tell them apart by measuring the signal decay.” By adding compensation methods to data analysis, he believes that cytometrists can further increase the number of parameters beyond what is currently possible.
Telford said, “There are going to be some interesting biological applications that can be done by looking at lifetime.”
According to Vacca, another benefit of measuring signal decay is the ability to eliminate background from endogenous fluorophores, known as autofluorescence. “It’s a nuisance for all experimenters, and it becomes worse the further into the UV region you go,” he said.
Laser solutions
Both Telford and Vacca credit the availability of a wide range of affordable lasers in the visible and UV regions for these advancements in flow cytometry. “The good thing about flow is that with the improved optics and detectors, you no longer need a lot of laser energy,” Telford said. “We now use relatively low-power lasers because the dwell time of the cell in the beam is short.”
Silicon Valley startups
Ann Russell, a senior applications engineer at optoelectronic giant OSRAM Opto Semiconductors, remembers when the lasers used in flow cytometry were predominately gas-ion tools and “as big as a college dorm room fridge.” She credits advancements in direct transmission and diode laser technology with helping to decrease the size of the instruments while increasing their quality. “Our single-mode beams are very clean,” she said. “They are exactly what our flow cytometry customers are looking for.” Improved waveguides, she added, have also contributed to beam quality.
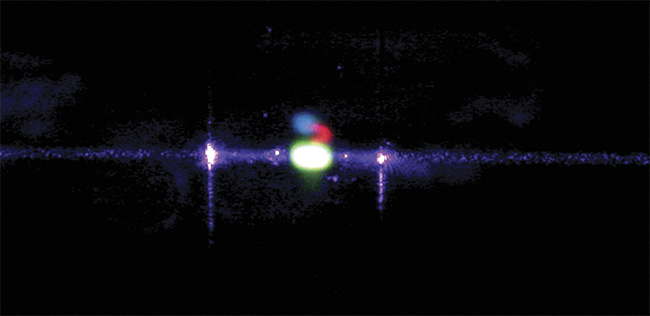
A fluorescein core stream in a flow cell illuminated by a 405-nm laser. The sample emits green
fluorescence, visible as the bright green spot. Courtesy of Kinetic River Corp.
Russell noted that on many of today’s lasers, researchers can adjust the temperature of the diode to allow up to a 15-nm wavelength variation. “This provides something extra, without having to go into the UV, which can cause ionization,” she said.
The combination of affordability and miniaturization could push flow cytometry into new areas, Russell said. In Northern California, where she is based, biotechnology startups are routinely using the technology. “You don’t have to outlay this huge investment that acts as a barrier to entry,” she said.
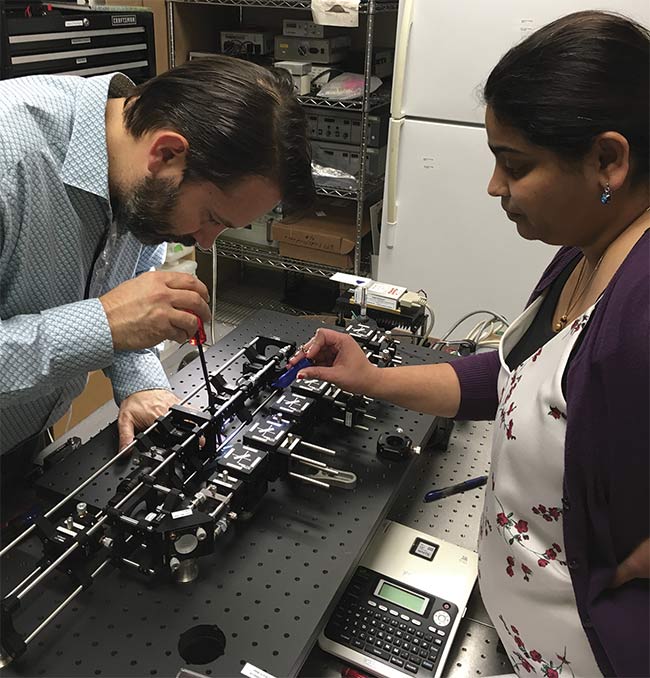
Giacomo Vacca (below left) and Kshitija Shevgaonkar of Kinetic River adjust a flow cytometer’s optics. With the emergence of improved optics and detectors, these instruments no longer need a lot of laser energy. Courtesy of Kinetic River Corp.
Telford said he has also seen an explosion in the growth of the field: “There are more instruments and more companies now than there ever have been.” Smaller, benchtop devices that can perform their own analyses will play a role in improving the health of individuals in remote parts of the world, he said. “A clinic in Kathmandu, for example, wouldn’t need to send perishable samples to a hospital in India.”
The data produced from the proliferation of these instruments will likely foster the use of advanced computational techniques in flow cytometry. “To have good artificial intelligence, you need lots of data to train it,” Russell said. “We humans are good at generating data.”
Telford also noted the trend toward improved data analysis in flow cytometry — spurred in part by the increase in the number of detectable parameters. “Whether it’s AI or machine learning, we’re starting to adopt the tools that the proteomics and genomics people have been using,” he said. “This will help us better visualize complex, multiparametric data and get results that we couldn’t get before.”
Nanoflow
Just as flow cytometers have been getting smaller, so too have their targets. “A very strong line of inquiry is in measuring submicron particles,” said Kinetic River’s Vacca. Submicron and nanoscale flow cytometry have taken advantage of the confluence of improvements in the technology, as well as the push to shorter-wavelength lasers.
John Tilton is an associate professor of nutrition at Case Western Reserve University, where he directs the immunobiology program at the university’s Center for Proteomics and Bioinformatics. Late last year, his lab reported using nanoscale flow cytometry to analyze the variability in activity of HIV protease in patients infected with the virus1. He said it was
the first time the technique had been used to directly measure enzymatic activity, and one of the first reported uses of nanoscale flow to look at patient-derived viruses.
Tilton has been using flow cytometry for more than 20 years and was interested in determining whether viruses, like cells, were heterogeneous. “When you see pictures of viruses in textbooks, they all look perfect,” he said. “But we know that they actually aren’t and wondered if we could uncover differences that have clinical implications.”
His group expressed viral genetic sequences taken from HIV-infected patients’ cells along with a reporter construct that contained a FRET (fluorescence resonance energy transfer) pair of fluorescent labels separated by an HIV protease cleavage site. The researchers stimulated the constructs with a 488-nm wavelength laser and developed a technique called fluorescence thresholding that identifies low levels of fluorescence, which would have been previously dismissed as background, to detect changes in emission. Only active proteases expressed from the viral sequences could cleave the construct efficiently, which quenched the fluorescence resonance energy transfer between the labels. Mutant enzymes did not have this ability.
Tilton discovered that the heterogeneity
in HIV protease activity mirrored the infectivity of those viruses — as well as their resistance to protease inhibitors, which are one of the main forms of HIV treatment. He further noted that, because viruses are so small, laser beam width, among other technical concerns, must be optimized for nanoscale flow cytometry. But he believes that the technique will become almost as routine as cellular analysis. “It’s really incredible how sensitive it is,” said Tilton, whose lab is in the process of building its own instrument.
“I see a big role for nanoscale flow cytometry in terms of understanding viruses and immunology better,” he said. “This has implications for vaccines, gene therapies, and viral treatments for cancer, which are based on viral particles.”
Telford agreed. He said, “It’s an exciting time to be in the field because there’s a lot of new instruments and technology being developed.”
www.linkedin.com/in/farooqtheahmed
Acknowledgments
The author would like to thank William Telford, the National Cancer Institute; Giacomo Vacca, Kinetic River; Ann Russell, OSRAM Opto Semiconductors; and John Tilton, Case Western Reserve University.
Reference
1. M. Bonar et al. (2020). Nanoscale flow cytometry reveals interpatient variability in HIV protease activity that correlates with viral infectivity and identifies drug-resistant viruses. Sci Rep, Vol. 10, Article No. 18101.