Tiny Silicon Chip Provides Broadband Gain at Telecom Wavelengths
Four-wave mixing provides frequency conversion as well as gain.
Breck Hitz
Although gain at telecom wavelengths has been demonstrated in silicon-on-insulator photonic circuits, until now the effect had depended on stimulated Raman scattering. The Raman bandwidth is relatively narrow, but modern optical telecommunications is based on wavelength multiplexing, with many signals, each at a different wavelength, carried over an optical fiber. Raman amplification cannot amplify all the wavelengths traveling on a given fiber.
Recently, however, researchers at Cornell University in Ithaca, N.Y., demonstrated broadband optical gain based on four-wave mixing in silicon. Unlike Raman scattering, four-wave mixing can simultaneously amplify all the channels that might be present on an optical fiber. Four-wave mixing is a nonlinear effect in which two pump photons are converted to one signal and one idler photon, such that energy is conserved (Figure 1). Four-wave mixing has been demonstrated in fibers not only for gain but also for other telecommunications functions such as all-optical switching and optical signal regeneration. However, these applications require many meters or kilometers of fiber.

Figure 1. In degenerate four-wave mixing, the energy in two pump photons is converted to a signal photon and an idler photon. In the silicon waveguide, four-wave mixing amplified the incoming signal so that a stronger signal exited the waveguide.
Because the nonlinear effect is 200 times greater in silicon than in silica glass, and because the silicon waveguides confine light ∼100 times more tightly than fibers do, the Cornell research offers the possibility of providing all these functionalities in tiny silicon chips that integrate both photonic and electronic capabilities.
Although conservation of energy in four-wave mixing is assured by the wavelengths of the pump, idler and signal photons, conservation of momentum — that is, phase-matching — is more complex. The researchers calculated that a key requirement is that the group-velocity dispersion be anomalous; that is, that the change in refractive index with frequency get larger with increasing wavelength. Unfortunately, the dispersion of bulk silicon is highly normal (nonanomalous) at telecom wavelengths. However, the effective index (and dispersion) experienced by light traveling in a waveguide is determined by two factors: the material’s bulk index and the effect of the waveguide itself.
The researchers calculated the effective dispersion of light at telecom wavelengths traveling in different-size silicon waveguides and found that, in some cases, the waveguide dispersion was great enough to pull the effective dispersion into the anomalous region (Figure 2A). In these cases, phase-matching was possible, and the model-predicted gain could be observed from four-wave mixing (Figure 2B).
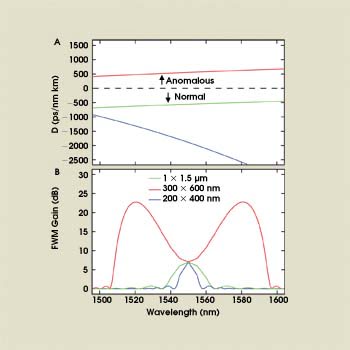
Figure 2. Because the total refractive index of light in a waveguide is the sum of the material’s bulk index plus the index caused by the waveguide itself, the dispersion can be greatly influenced by waveguide design, as seen in (A). The researchers’ model predicted that anomalous dispersion — which is necessary for phase-matching in four-wave mixing — could be achieved in a waveguide with a 300 × 600-nm cross section. But in a larger (1 × 1.5 μm) or smaller (200 × 400 nm) waveguide, the dispersion is normal, and four-wave mixing cannot be phase-matched. In (B), the model predicted that gain caused by four-wave mixing (FWM) was possible in the 300 × 600-nm waveguide, but not in the other two.
Experimentally, the researchers fabricated silicon-on-insulator waveguides with a 1-μm buried oxide layer and pumped them with femtosecond pulses at 1550 nm. They probed the waveguide’s gain with femtosecond signal pulses tunable between ∼1510 and ∼1590 nm. (The telecom C-band is nominally defined as 1530 to 1565 nm.) They observed gain across differing bandwidths, depending on the waveguide’s geometry (Figure 3). The experimental gain was less than that predicted by the model in Figure 2 because of nonlinear absorption, free-carrier absorption and pump depletion.
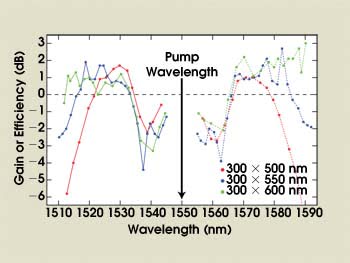
Figure 3. Experimentally, the researchers observed gain from four-wave mixing over various spectral regions, depending on waveguide geometry.
Other experiments conducted at Cornell and elsewhere have shown successful techniques for minimizing these effects, and the researchers expect that they could obtain significantly better results by integrating these techniques into their experiment.
Wavelength conversion is another important function in telecommunications; if an outgoing channel at a particular node is using the same wavelength as an incoming channel, the incoming channel would have to be converted to another wavelength. Currently, this effect is achieved by an optics-to-electronics-to-optics conversion. The incoming optical signal is detected and converted to an electrical signal, which in turn drives a laser at a different wavelength. The ability to perform all-optical wavelength conversion would greatly simplify the system. Because four-wave mixing can not only amplify the signal wavelength, but also produce a new signal at the idler wavelength, it can provide this functionality.
Nature, June 22, 2006, pp. 960-963.
Published: September 2006