With terahertz imaging systems getting smaller and cheaper – and performing better – applications are stacking up in cancer imaging as well as drug detection and development.
Terahertz spectroscopy is a fast-growing area of research with some hugely promising applications, now that cumbersome, expensive and hard-to-use systems are in the past. Its low-photon-energy radiation makes it safe for tissue imaging, and its high absorption in water, which is often a key indicator of the presence of tumor cells, makes it a hopeful weapon in fighting cancer.
Revealing tumors in more detail
Cancer is the second most common cause of death in the US, second only to heart disease, and accounts for nearly 1 in every 4 deaths, according to the American Cancer Society. Early detection could mean the difference between life and death for many patients, but there is a shortfall in the current imaging techniques.
X-ray imaging and MRI provide images of living tissues at the macroscopic level, but with low resolution and specificity. Microscopic imaging can be used on biopsy samples to provide structural and functional information, but there is not yet a technique that can noninvasively image tissue at a high resolution – effectively combining both macroscopic and microscopic imaging.
New terahertz spectroscopic studies of cancer could potentially fill this niche. Since terahertz frequencies are readily absorbed by water and other polar liquids, the method lends itself well to imaging most organic tissue.
Although the high absorption loss limits the depth of imaging, it does promote extreme contrast between substances with lesser or higher degrees of water content, which helps to show distinctive contrast in medical imaging.
“High absorption of water and other polar liquids in terahertz frequency range limits the sensitivity of terahertz imaging in water-rich samples,” said Dr. Trung Quan Luong of the Applied Competence Cluster Terahertz at Ruhr University Bochum in Bochum, Germany. “The focus of current biomedical application is on skin imaging. Further improvements require high-power terahertz sources.”

(Left) Outside and inside a package of omeprazole. (Right) Terahertz image of the packaged omeprazole showing that the terahertz beam can penetrate the paper and plastic packages to observe the inner drug content. Courtesy of Applied Competence Cluster Terahertz, Ruhr University Bochum.
In a bid to further improve image contrast, Korean scientists introduced gold nanoparticles to label cancer cells (Optics Express 2009;17:3469-75). In a paper reviewing various forays into terahertz imaging, published in March 2012, professor Emma MacPherson at the Chinese University of Hong Kong and colleagues explain the innovative approach in more detail.
Gold nanorods (GNR) are engineered to be readily absorbed by cancer cells and injected into the specimen. At first, terahertz imaging reveals nothing, but once an IR laser is used to heat the nanoparticles, a notable increase in reflected terahertz signal is detected. Interestingly, since the positioning of IR light illumination can be controlled with micron resolution, the image resolution of the terahertz-differential image measurement can also be on the order of microns – much higher than with conventional terahertz imaging.
What’s more, with high enough concentrations of GNRs and intense exposure of near-IR light, cells can be destroyed. This means that if the GNRs can target the cancer cells with high enough specificity, the technique also has potential for hyperthermia therapy for cancer.
But MacPherson is quick to point out that further research into the safety of the technique needs to be fully investigated first.
“In terms of developing terahertz imaging/spectroscopy into a clinical technique for cancer diagnosis, there is still a long way to go,” MacPherson said. “More studies and clinical trials are needed to determine whether terahertz imaging has significant advantages over existing techniques.”
At National Taiwan University, Dr. Chi-Kuang Sun and colleagues demonstrated in vivo breast cancer detection in a mouse model for the first time. The group used a terahertz-fiber-scanning transmission imaging system to continuously monitor the growth of human breast cancer in mice. Cancer cells were first implanted into the mice; then continuous screening distinguished cancer development from the surrounding fatty tissue.
“Early cancer development was detected on the twenty-third day, and the induced terahertz absorption coefficient change was 0.25 mm−1, corresponding to a cancer volume of 1.3 mm3,” Sun said. “This change is mainly due to the change in the water content in these tissues.”
While this study indicates the potential for noninvasive early cancer detection with high sensitivity and without the
need for labeling, it does not prove that this imaging system can be directly applied to humans in vivo.
The problem is enhancing the image contrast of cancerous cells buried within fibrous breast tissue rather than fatty breast tissue. In addition, gland tissue – which also has high water content – shows up with similar contrast to cancerous tissue under terahertz illumination. Since breast tissue can be fatty and originates from glandular tissue, the potential of this technology could be seriously limited for human screening.
One possible solution is to perform time-lapsed imaging to provide a base from which cancerous tissue can be distinguished from other gland tissues, but Sun admits that further work still needs to be done.
Although progress is being made in many areas, the competition from more developed imaging modalities is fierce. Ultrasound, OCT, near-IR and Raman spectroscopy, MRI, positron emission tomography, in situ confocal microscopy, and x-ray techniques have all received much more attention and currently offer enhanced resolution, greater penetration, higher acquisition speeds and specifically targeted contrast mechanisms.
But terahertz imaging is catching up fast and offers some elements that existing techniques do not. For example, no technique can readily distinguish benign from malignant lesions macroscopically at the surface or subdermally. Furthermore, the sensitivity of terahertz signals to skin moisture (which is often a key indicator) is very high, and competing techniques such as high-resolution MRI are less convenient and more costly.
Drug detection, development
Today, more terahertz systems are commercially available than ever before – and with more competition come many improvements.
“Advances in recent years have been on the source and detector side,” said Dr. David Armstrong, marketing director at M Squared Lasers in Glasgow, Scotland. “At M Squared, we have developed a turnkey tunable terahertz source, the Firefly-THz, using licensed technology developed at [the University of] St. Andrews [in Scotland] – namely, a pulse OPO [optical parametric oscillator] approach that yields high peak power and narrow linewidth, suitable for standoff imaging and resolving spectral components.”
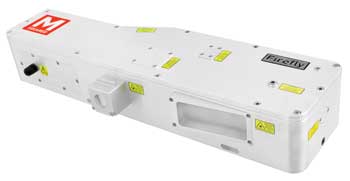
M Squared Lasers’ Firefly-THz is a hands-free, pulsed nanosecond terahertz laser source that provides a tunable narrow-linewidth output, thanks to its novel optical parametric oscillator design. Courtesy of M Squared Lasers.
Building on 15 years of experience in developing parametric generators for the UV-VIS-IR spectral ranges, researchers at St. Andrews have developed a compact, efficient and transportable parametric generator of terahertz radiation.
The system has the potential to identify
The system has the potential to identify and characterize illegal drugs that are concealed about a person, sent through the mail or on a contaminated surface. Unlike x-ray scanners, canine detection or external swiping, terahertz waves can noninvasively penetrate a wide range of materials such as paper, wood, plastic, fabrics, ceramics, bone and tissue.
Not only can terahertz imaging detect a concealed drug, but it can also provide a spectral fingerprint, making drug identification possible.
Advances in 3-D terahertz imaging are opening up new avenues in pharmaceutical applications, where it can improve the quality and uniformity of pharmaceutical products. Many pharmaceutical materials can exist in multiple solid forms, known as polymorphic forms: They have the same chemical composition but different crystalline structures and as such behave differently in terms of solubility and stability.
Terahertz spectroscopy can pinpoint at which step in the manufacturing process the polymorphic transitions are emerging, giving pharmaceutical makers a better understanding of the causes of changes in structure.
But it’s not just what’s on the inside that matters; the tablet coating is also a crucial component that impacts how the drug interacts with our body. For oral drugs, the coating protects the active ingredients from being released before the tablet reaches the small intestine, where they are absorbed.
Coatings can also prolong a tablet’s shelf life by protecting the components from moisture and oxygen degradation. Therefore, characterizing the tablet coating and ensuring uniformity within a single tablet or an entire batch is important.
Here, terahertz pulse imaging measures the thickness of coatings by a change in refractive index of reflected light. Although the technique provides results similar to ultrasound measurements, there remains one common practice in the pharmaceutical industry in which terahertz triumphs.

The interaction of a terahertz pulse with a protein and its hydration shell. Courtesy of Trung Quan Luong, Konrad Meister and Martina Havenith, Ruhr University Bochum.
Terahertz data compiled from measuring the coating thickness has been found to strongly correlate with the dissolution profile of the tablet. Determining how a drug dissolves is currently performed using a dissolution apparatus, often requiring lengthy time analysis. Terahertz, on the other hand, offers a promising way to reduce the cost and time involved.
In terms of pharmaceutical applications, extending the spectral range of current instruments from 100 to 300 cm−1 would allow coverage of both the intermolecular and some of the intramolecular vibration modes of pharmaceuticals. This would not only provide better spectral specificity for chemical discrimination and mapping, but would also help to characterize thinner coatings with higher spatial resolution.
References
1. C. Yu et al (March 2012). The potential of terahertz imaging for cancer diagnosis: A review of investigations to date. Quant Imaging Med Surg, Vol. 2, pp. 33-45.
2. American Cancer Society Cancer Facts & Figures 2011. American Cancer Society, Atlanta. Available online at http://www.cancer.org/Research/CancerFactsFigures/CancerFactsFigures/cancer-facts-figures-2011.
3. L.M. Ingle et al (2013). Terahertz spectroscopy for pharmaceutical applications. Int J Pharm Pharm Sci Res, Vol. 3, pp. 48-52.
Top bio-related applications of terahertz spectroscopy
• Tumor imaging
• Characterization of peptides,proteins, DNA, RNA, etc.
• Burns/wound assessment
• Dentistry
• Drug detection and identification
• Pharmaceutical coating and content characterization
• Food process monitoring and quality control (such as moisture determination, oil characterization, foreign body detection, package inspection and beverage characterization)