As consumer and enterprise demand for faster computers increases, so will the demand for faster computer chips. The speed of computer chips is directly related to the number of transistors that can fit on a chip.
Transistors work like switches that enable and stop the flow of electricity, which corresponds directly to the binary code of ones and zeroes that underlies all computer functions. The more transistors, the more zeroes and ones are available to make more elaborate codes.
In 1965, Gordon Moore predicted that the number of transistors that can fit on a computer chip will double every two years. This prediction has become known popularly as Moore’s Law, and it has been true for 40 years, thanks largely to Intel, the company that Moore founded with Robert Noyce, which is still the world’s largest chip maker. However, physical limitations may prevent Moore’s Law from continuing to be true.
Transistors are assembled in recessed areas on a chip. Thus, to create more transistors, smaller and smaller recessed areas must be created in a process called photolithography, which is accomplished by shining light uniformly on a mask – a light-blocking material with geometrically patterned spaces cut into it.
The fineness of the spaces in the mask limits all but very small wavelengths of light from passing through. Currently, 193-nm deep-ultraviolet light is used, but researchers want to use extreme-ultraviolet (EUV) light of 13.5 nm to penetrate even finer spaces so that they can assemble even more transistors on computer chips. The light sources of extreme-ultraviolet are the limiting factor in this endeavor.
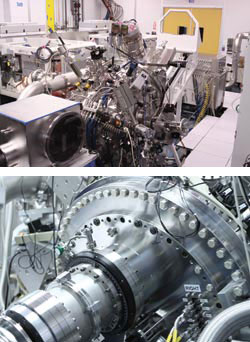
Shown above are two views of the EUV source that was shipped from Cymer to ASML for its beta-level EUV lithography scanner. Courtesy of Cymer.
“EUV sources have come a long way from being a lab curiosity,” said Vivek Bakshi, the president and founder of EUV Litho Inc. of Austin, Texas, through which he offers consulting services, workshops and an annual meeting in Hawaii on EUV technology.
“Today, multiple suppliers around the world … are investing lots of money and effort to make sources that can support high-volume chip making – the stage at which you are making products to sell,” Bakshi added.
Inside an EUV source
EUV sources are big, shiny, metal machines that attach onto other big, shiny, metal machines referred to as scanners. The computer chips are placed in these scanners, and EUV light from the sources is routed to the scanners using collector optics such as special mirrors that reflect EUV.
There are two types of power sources: laser-produced plasma and electric discharge-produced plasma. Because the laser sources are significantly more powerful than the electric sources, they are being developed for beta-level scanners, whereas electric sources were used in alpha scanners.
Bakshi said that Netherlands-based ASML and Tokyo-based Nikon are the leading makers of EUV lithography scanners, and ASML is ahead of Nikon with a beta-level scanner in production.
Laser-produced plasma EUV sources are fabricated by firing a rapidly pulsed infrared laser at molten droplets of tin. These tin atoms can contaminate the computer chips, so a debris filter is necessary. “Integrating an EUV source with a collector and a debris filter to make a system known as a source collector module is a big challenge,” Bakshi said.
The goal for a completely integrated beta-level system is about 60 to 100 W of power. A San Diego company called Cymer has provided a laser-produced plasma EUV source for ASML that, in a press release, it said was measured at 75 W.
However, Bakshi said that the power level is meaningful only if the source is integrated completely with the scanner, for the reasons stated above, and the power level must be continuously stable for a long time to meet the demands of industrial-scale production.
David Brandt, senior director of EUV development at Cymer, told Photonics Spectra that the 75-W power from its EUV source was measured on a development system in its facility using a NIST-calibrated EUV photodiode to record the plasma emission through a zirconium foil.
The EUV source was operated at 40 percent duty cycle in 400-ms bursts – long enough to expose a full 32-mm field, assuming a 10 mJ/cm2 resist. The power was run for the course of a day while the parameters were varied to determine the best ones. Once the best parameters were determined, the Cymer engineers ran it for an hour or so.
Alternatives to EUV
EUV is not the only way to break the photolithography limit. The wavelength could be shortened using lenses such as the plasmonic superlens developed by the Xiang Zhang group and put on a flywheel with the assistance of the David Bogy group, both at the University of California, Berkeley, as described in the December 2008 issue of Nature Nanotechnology.
Another strategy that could work involves the use of self-assembling polymers such as those described by Thomas Russell of the University of Massachusetts Amherst and Ting Xu of Berkeley in the Feb. 20, 2009, issue of Science.
Some scientists have described the use of lasers and other light sources to shape acrylic polymers, which are used frequently as photoresists on computer chips. Similar techniques based on this premise have been developed independently by the groups of Robert McLeod at the University of Colorado at Boulder, John Fourkas at the University of Maryland and Rajesh Menon at MIT.
“As opposed to EUV techniques, ours can be conducted in ambient atmosphere rather than under vacuum conditions,” Fourkas said. “In addition, it is far easier to work with near-infrared light than EUV, and the optics are far less expensive.”
All three methods are detailed in the May 15, 2009, print issue of Science. According to the papers, the inspiration for all of the techniques was stimulated emission depletion microscopy, in which fluorescent molecules are excited by a fluorescent laser pulse, and then a second laser pulse of a substantially longer wavelength is used to de-excite the molecules through stimulated emission.
Essentially, all three groups used an acrylic resin mixed with a dye and shined two laser beams on the mixture. One beam caused the resin to harden in certain areas, while the other beam prevented hardening.
The Menon and McLeod groups used UV and visible light, and the Fourkas group used two infrared Ti:sapphire lasers, one pulsed and the other always on. The McLeod group also used LED and mercury lamp light sources, which are cheaper than lasers.
According to McLeod, “The main advantage of our technique is that it uses two single-photon processes. That means we can potentially scale to commercially interesting throughput. Two-photon absorption is very, very inefficient ….”
The Fourkas technique is called RAPID (resolution augmentation through photo-induced deactivation). “RAPID was chosen as an easy-to-remember acronym, but it is a serial technique currently and therefore is not tremendously fast,” Fourkas said. “We are working on a considerably more parallel version,” he added.
Fourkas continued, “RAPID offers significant advantages over [the McLeod] technique for 3-D fabrication and also only requires a single color of light. In addition, the photo-initiator is regenerated in RAPID, whereas in [the McLeod] technique, both the initiator and inhibitor are used up upon exposure. Under some conditions, this can lead to difficulties in fabrication, particularly in thicker samples. However, their method is very good for 2-D fabrication in thin samples.”
Industrial scale
Although Bakshi is interested in all of these alternatives to EUV, he is skeptical about any of them achieving industrial-scale throughput. He pointed out that, once it is developed, EUV can be adapted to existing systems, but that the other techniques probably will need more extensive adaptation to existing industrial systems. He added that the technology needs to come out at the right time. For instance, 157-nm photolithography was invented but never caught on because of poor timing.
Just what is the current volume of wafer processing at industrial speeds? “Currently, a lithography scanner needs to print at least five hundred 300-mm wafers per hour,” Bakshi said. “This means it takes about 36 seconds to print one wafer.” The Intel dual-core Atom processor, for instance, contains 47 million transistors. Imagine printing one of these chips with 47 million transistors in 36 seconds.
Ultimately, time will tell which of these methods gets adapted to industrial-scale lithography. This area of research and development is extremely competitive. May the best technology win.