Researchers led by Nobel laureate Stefan Hell at the Max Planck Institute for Medical Research have developed a superresolution microscope with a spatiotemporal precision of 1 nm/ms. The work builds upon the team’s recently introduced MINFLUX superresolution microscopy technology, now enabling users to observe the miniscule movements of single proteins at what the researchers called an unprecedented level of detail.
Investigating the inner workings of a cell requires knowledge of the biochemistry of individual proteins, the key challenge being the measurement of the minute changes in their position and shape. Fluorescence microscopy, particularly superresolution microscopy, is vital in this field. MINFLUX has so far attained a spatial resolution of one to a few nanometers: the size of small organic molecules. Understanding molecular cell physiology further requires observations at even higher temporal resolution.
MINFLUX was originally used to track fluorescently labeled proteins in cells. Their movements, however, were random and the track had precisions of the order of tens of nanometers. In its current work, the team applied the resolving power of MINFLUX to conformational changes of proteins, specifically the motor protein kinesin-1.
The newly introduced version of MINFLUX can be used to track single fluorescent molecules.
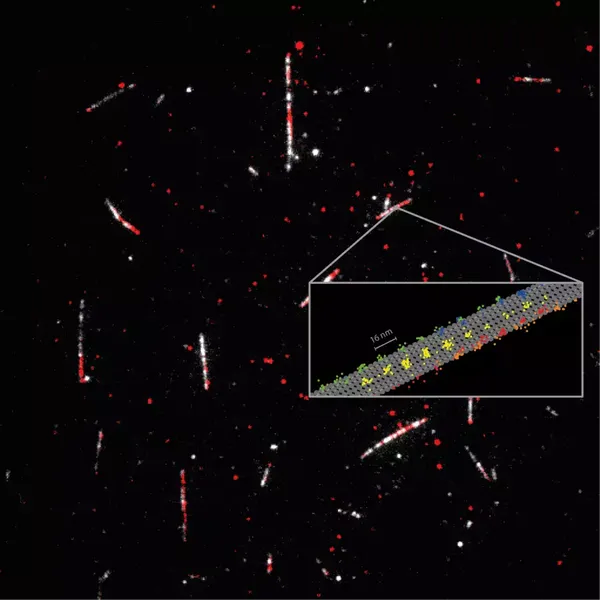
Detailed measurement of how the motor protein kinesin-1 (red) walks on microtubules (white). Tracking of the 2D movements of individual kinesin-1 dimers (color coded on the sketch) at physiological ATP concentrations revealed key details of how the protein walks in individual lanes. MINFLUX facilitated near protofilament tracking of the motor protein on the microtubule (sketched in gray). Courtesy of MPI for Medical Research.
Established methods for measuring protein dynamics have severe limitations that prevent them from addressing the subnanometer and submillisecond range. Some provide a high spatial resolution, down to a few nanometers, but cannot track changes fast enough. Others have a high temporal resolution but require labeling using beads that are two to three orders of magnitude larger than the protein being studied.
MINFLUX, however, requires only a standard 1-nm-size fluorescence molecule as a label attached to the protein, and therefore can provide both the resolution and the minimal invasiveness that are needed in studying native protein dynamics.
“One challenge lies in building a MINFLUX microscope that works close to the theoretical limit and is shielded against environmental noise,” said Otto Wolff, a Ph.D. student in the research group.
“Designing probes that do not affect the protein function, but still reveal the biological mechanism, is another,” said postdoctoral researcher Lukas Scheiderer.
The updated version of MINFLUX presented in the study can record protein movements with a spatiotemporal precision of up to 1.7 nm/ms. It requires the detection of only about 20 photons emitted by the fluorescent molecule.
“I think we are opening a new chapter in the study of the dynamics of individual proteins and how they change shape during their functioning,” Hell said. “The combination of high spatial and temporal resolution provided by MINFLUX will allow researchers to study biomolecules as never before.”
Kinesin-1 is used to transport cargo throughout cells, and mutations of this protein are at the heart of several diseases. Kinesin-1 moves in a walking motion along the microtubules that connect cells. The movement typically occurs along one of the 13 protofilaments forming the microtubule, and it is fueled by the splitting of the cell’s principal energy supplier, ATP (adenosine triphosphate).
Using only a fluorophore for labeling the kinesin-1, the researchers recorded the 16-nm steps of the protein, as well as 8-nm substeps, with nanometer per millisecond spatiotemporal resolution.
The results proved that ATP is taken up as a single “head” of the protein bound to the microtubule, but that ATP hydrolysis occurs when both heads are bound. It also revealed that the stepping involves a rotation of protein “stalk,” the part of the kinesin molecule that holds the cargo, as well as a rotation of the head in the initial phase of each step.
The research was published in Science (www.science.org/doi/10.1126/science.ade2650).