Optical fibers deliver laser light that helps image the severity of arteriosclerosis, break apart plaques with ablation, and heal damaged tissue after surgery.
Patients with heart disease often face a daunting journey from diagnosis to treatment. They may learn, after experiencing chest pains or shortness of breath, that they have arteriosclerosis, or damaged arteries, through a test such as a coronary angiogram, which shows via x-rays whether blood vessels are restricted. In extreme cases, coronary artery bypass surgery is performed, which is a highly invasive procedure with a lengthy recovery time. But due to the specificity made possible by laser-based techniques, shorter and more effective diagnostics and therapeutics are becoming increasingly viable in clinical settings.

A fiber red laser ablates a plaque to cross a chronic total occlusion inside a microcatheter using tiny pull wires. Courtesy of Clayton Foundation for Research.
Heart disease has long been the leading cause of death in the U.S., costing 697,000 men and women their lives in 2020, according to the Centers for Disease Control and Prevention (CDC). The most common form of heart disease is coronary artery disease, which is the result of plaque buildup — composed of cholesterol and other deposits — that narrows the arteries over time, restricting blood flow to the heart. Approximately 382,820 U.S. citizens died from coronary artery disease in 2020, and millions more were afflicted with the condition, the CDC reported.
The American Heart Association attributes these alarming statistics to a number of health and lifestyle behaviors: Smoking, sedentary living, and a poor diet lead to high blood pressure and elevated glucose and cholesterol. To make matters worse, many of these behaviors have only risen among people who have limited access to medical care.
This depressing reality has galvanized the expansion of solutions in laser-based cardiology research at numerous institutions, as well as inspired innovation at companies whose systems have been applied in experiments that go beyond traditional protocols, such as the administration of pharmaceuticals. Lasers were employed to destroy arterial plaques at the Oregon Medical Laser Center in the 1990s. Since then, the advancement of endoscopic imaging and catheterization of surgical tools has improved the precision of laser delivery, as optical coherence tomography (OCT) and other imaging modalities have guided the treatment to the source of the problem. This trend accelerated with the U.S. Food and Drug Administration’s clearance of the cardiovascular technology produced by Ra Medical in 2017, through which laser therapy could be delivered through a liquid core catheter. This has continued with recent research regarding the use of excimer laser coronary angioplasty, which uses lasers to create a vapor bubble that breaks up plaques. Researchers at the University of Kansas, Washington University at St. Louis, and elsewhere believe that, in time, this could create a fundamental change in clinical protocols for the treatment of atherosclerosis.
The utility of mid-IR lasers
The need to identify and treat arterial plaques has affected the types of lasers that have found their way into the commercial market. Several companies, such as DRS Daylight Solutions, develop quantum cascade lasers (QCLs) that take aim at plaques and other markers of cardiovascular health. The lasers were first developed in 1994 and function at wavelengths in the mid-IR range, between 2500 and 25,000 nm. In this range, lasers provide coherent and polarized light in continuous-wave or pulsed form, with the advantage of spectral repeatability, and they are aligned to capture the chemical fingerprint of specific molecules, such as glucose, proteins, and lipids.
“It really depends on how you want to use the QCL,” said Jason Sorger, a senior field service engineer with DRS Daylight. “For example, lasers in this range have been shown to be effective in the identification of atherosclerosis with photoacoustic microscopy, and you need really high-quality laser light for that application.”
In a recently published study, a pulsed quantum cascade laser, the MIRcat from DRS Daylight, was used in mid-IR optoacoustic microscopy. Laser pulses are absorbed into tissue, which causes thermal expansion and the release of ultrasonic waves that reveal structural details. This effect helps determine the composition of plaques in human carotid artery samples, which includes cholesterol, carbohydrates, lipids, and proteins (Figure 1). In a system used for this experiment, the quantum cascade laser provided the optoacoustic signal, which was detected by a 20-MHz ultrasound transducer that receives the echoes created, and the results were digitized using data-acquisition software1.
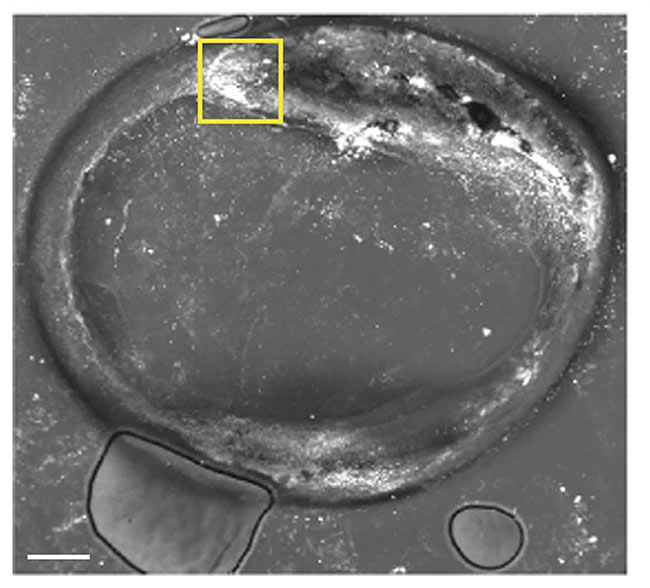
Figure 1. A mid-infrared optoacoustic microscopy image of the features of a plaque deposit at 2850 cm−1. Adapted with permission from Reference 1.
For this type of analysis to be practical in a clinical setting, according to Sorger, the lasers must provide spectral repeatability, or hit the desired spectral range every time a reading is sought, which requires precise tuning. Tunable mid-IR lasers are useful in glucose monitoring, capturing the specific spectral details at a depth of up to 100 µm in a complex mixture of biomolecules after being integrated into a noninvasive glucometer that is attached to the skin2. Elevated levels of glucose can result not only in diabetes but also in cardiovascular disease.
While quantum cascade lasers provided by companies such as DRS Daylight have found a niche in research applications, to proliferate in medical diagnostics, they will need very high throughput, meaning the capture of reliable data at speeds necessary for swift medical diagnosis. Companies such as DRS are devising various ways to package the lasers for integration into common medical devices, Sorger said.
“It’s difficult to have high quality at high speed,” he said. “It is essential to have even power distribution and good illumination sources so you get better data that can be reproduced reliably each time the laser is fired.”
Lasers aimed at plaque buildup
Historically, doctors have used balloon angioplasty and implanted stents to treat blood vessels that are narrowed by plaque buildup. In most percutaneous coronary interventions, a wire is guided through an artery past the blockage point and a tiny balloon attached to a catheter is inflated to widen the artery opening to allow increased blood flow to the heart. A stent is then put in place to keep the vessel functional by holding it open. This procedure, while it can be effective, is not without limitations, and complications may eventually result.
“Over time, the stent can narrow, and scar tissue forms,” said Marc Sintek, an interventional cardiologist and associate professor of medicine at Washington University School of Medicine in St. Louis. “The composition of plaque buildup and scar tissue in the stent is complicated, but the laser can get past that structure by breaking up that hardened material.”
In excimer laser coronary angioplasty (ELCA), the plaque material absorbs fiber laser pulses that last only nanoseconds in the UV range, which provides the photons with the energy to break apart the molecular bonds. Water is then vaporized, creating a bubble that causes additional ruptures in the plaques. Equipment for ECLA is manufactured by Philips, and the system contains a unit that generates the laser beam that is delivered through a set of catheters of various sizes, containing numerous fibers surrounding a guidewire.
Two years ago, a study was conducted to determine the safety of ELCA using procedures that were reported to the National Cardiovascular Data Registry (NCDR) and the CathPCI Registry (the percutaneous coronary intervention registry) between 2009 and 2018. The risk of complications, such as perforation, remained low for treatments such as using ECLA to treat in-stent restenosis, or the gradual narrowing of the vessel after a stent is in place. In the case of chronic total occlusions, the risk was higher than average. According to Sintek, the higher risk was partially attributable to the compromised health of patients suffering from chronic total occlusions. The study authors acknowledged that ELCA should therefore be administered carefully on a case-by-case basis3.
Sintek partially attributed the low use of ELCA by medical professionals to the expense and complication of installing the laser system in a clinical setting. He said initial uses of the technology required a 240-V receptacle.
“But our current system can plug into any outlet,” Sintek said. “There are a number of procedures a cardiologist can use, but I look at it like a carpenter with a toolbox and having every tool available. Today, the hot topic is precision medicine, and lasers are enabling that precision in treating coronary artery disease.”
Honing the catheter method
A team of researchers from the Beckman Laser Institute and Medical Clinic at the University of California, Irvine, in collaboration with University of Texas Health, has been developing a catheter-based system to deliver a laser-induced shockwave to break up calcification in arteries. The system combines optical fibers that deliver 755-nm alexandrite laser pulses with a fluid-filled balloon that expands to about 2 mm to make contact with the arterial wall through a guided catheter. Various pulse durations were used in coronary artery phantoms to effectively fracture the calcium deposits.
“The balloon, in this case, is merely the conduit for the pressure waves to the area that is being treated,” said Nitesh Katta, a postdoctoral researcher at the Beckman Laser Institute. “It clears an acoustic path for the laser to induce fractures, breaking up the calcium deposits.”
The technology is called intravascular laser lithotripsy, and it is rooted in transferring laser energy to pressure waves involving light as opposed to electricity. The system can be guided using OCT, and it is generally used when the vascular network is partially occluded or blocked.
“The ultimate goal is to reestablish compliance in the artery and do it without a stent,” Katta said. Compliance refers to improving the arterial mechanical performance as it relates to improving blood flow to a particular part of the network. Alternative methods include rotational atherectomy, in which a rotating burr is sent through the artery, or electric discharge plasma mediated shockwave therapy, which uses electrical pulses to generate shockwaves that create cracks in calcium deposits. But the latter treatment must be used sparingly because it runs the risk of disrupting the cardiac rhythm.
The term laser lithotripsy is derived from its common use in urology to break up kidney stones. And with the integration of industrial thulium fiber lasers (either femtosecond or picosecond), its utility has expanded to include potential applications in cardiology (Figure 2). Katta was awarded a research grant from the American Society for Laser Medicine and Surgery for this work, which began with the use of a preclinical animal model.
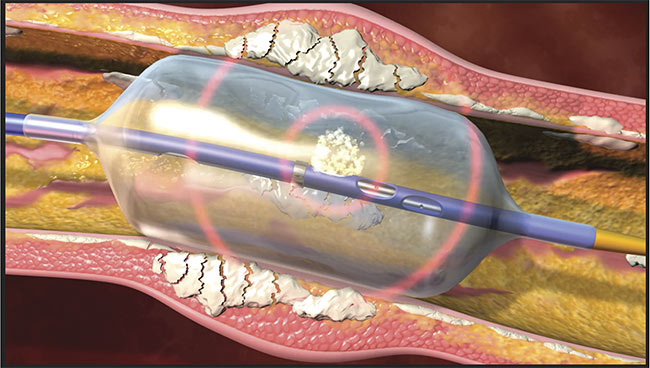
Figure 2. A fiber laser delivered through a catheter breaks up calcium deposits in arteries. Courtesy of Clayton Foundation for Research.
The group has also been exploring the utility of lasers for cutting through chronic total occlusion, which often has a cap of calcified material with softer lipids underneath. Traditional treatments include pharmacological treatments and coronary artery bypass, but the former does not treat the underlying causes of blockage and the latter is highly invasive.
In response, the UC Irvine group, in collaboration with University of Texas Health, constructed a catheter system, which was also guided by OCT and that contained a fiber-coupled holmium laser that fired 200-µs pules (see first image in article). The laser worked alongside a 200-µm conduit that fed CO2 via a pump mechanism for cooling. An IR camera was used to image the laser-irradiated region. This technique worked in a series of ex vivo samples, as well as in an in vivo rabbit femoral model. According to the team, the technique could be improved by limiting the volume of CO2, or through the use of chilled saline needled through a valve at the catheter tip, which could be guided by advanced imaging techniques for improved maneuverability.
“Occlusion typically occurs in ‘bends’ of the arteries, so it’s important we have maximum flexibility in the tip,” Katta said. “We are looking at the use of photoacoustic imaging and other techniques to guide the procedure.”
Sealing the deal
The role of lasers in both diagnosis and treatment can be integrated with other photonic technologies, such as in the sealing of blood vessels following surgeries to treat cardiovascular disease. A team at the University of North Carolina at Charlotte (UNCC), for example, has developed a system that uses an IR laser to seal blood vessels, and it subsequently uses UV light to monitor the fluorescence that displays whether the seal has taken.
The UNCC researchers simulated a potential future medical protocol by utilizing a 1470-nm diode laser to cut and seal blood vessel models at power levels of <25, ~100, and 200 W. Beam profiles and seal zones were carefully analyzed along with blood pressure. The 1470-nm wavelength is ideal because it parallels the wavelength absorption of water, which compressed blood vessels mostly consist of. Sealing procedures have historically been accomplished with radiofrequency and ultrasonic devices, but the laparoscopic device jaws need time to cool between applications. Experiments performed with porcine blood vessels have shown the IR laser to be an effective tool4.
The group has also designed a transparent quartz laparoscopic jaw with a side-firing 110-W, 1470-nm fiber laser attached to a servo motor. A thermal camera and micro-thermocouples were used to monitor temperature changes in the tissue while laser sealing was tested on 20 ex vivo vessel samples through 550-µm core fibers. An LED was used with fibers connected to a spectrometer with a long-pass filter to establish the presence of fluorescence that was indicative of a proper seal5.
Nathaniel Fried, a UNCC professor in the Department of Physics and Optical Science, directed the research. Successful seals were accomplished at 30 W for 5 s with the 1470-nm laser, and shorter seals of 1 s at higher powers have been previously demonstrated as well, he said.
The researchers said they will focus future efforts on producing a handheld device for sealing and cutting in animal models.
“For next steps, we would like to integrate the optical feedback systems, diffuse optical transmission and/or fluorescence, into a standard 5-mm-OD, Maryland-style, laparoscopic jaw,” Fried said. “We would also like to create a fully automated system, with closed-loop feedback to immediately deactivate the laser once we have achieved a successful seal.”
References
1. M. Visscher et al. (2022). Label-free analytic histology of carotid atherosclerosis by mid-infrared optoacoustic microscopy. Photoacoustics, Vol. 26, No. 100354.
2. T. Lubinski et al. (2021). Evaluation of a novel invasive blood glucose monitor based on mid-infrared quantum cascade laser technology and photothermal detection. J Diabetes Sci Technol, Vol. 15, No. 1, pp. 6-10.
3. M. Sintek et al. (2021). Excimer laser coronary angioplasty in coronary lesions: use and safety from the NCDR/CATH PCI Registry. Circ Cardiovasc Interventions, Vol. 14, No. 7, p. e010061.
4. N. Giglio and N. Fried. (2021). Sealing and bisection of blood vessels using a 1470 nm laser: optical, thermal, and tissue damage simulations. Proc SPIE, Vol. 11621.
5. N. Giglio et al. (2022). Reciprocating side-firing fiber for laser sealing of blood vessels. Proc SPIE, Vol. 11936.
Microlasers Target Heart Cells During Contraction
While high-speed imaging methods to capture the beating heart are readily available, measuring contractions deep within scattering cardiac tissue is still a huge challenge. A spectroscopic technique developed at the University of Cologne is changing that.
Researchers have applied whispering gallery mode (WGM) micro- and nanolasers to interact with live cardiac cells, which they found provide accurate spatial and temporal information about heart dynamics during the process of contraction (see figure). The basic principle behind WGM lasers is that when energy is pumped into a tiny sphere, the resulting emission pulse gains strength, similar to how audio waves move in a dome. The lasers have the capacity to detect minute changes of cardiomyocytes, the cells responsible for the heart contraction. This capacity has the potential to advance the research and therapy of heart defects.
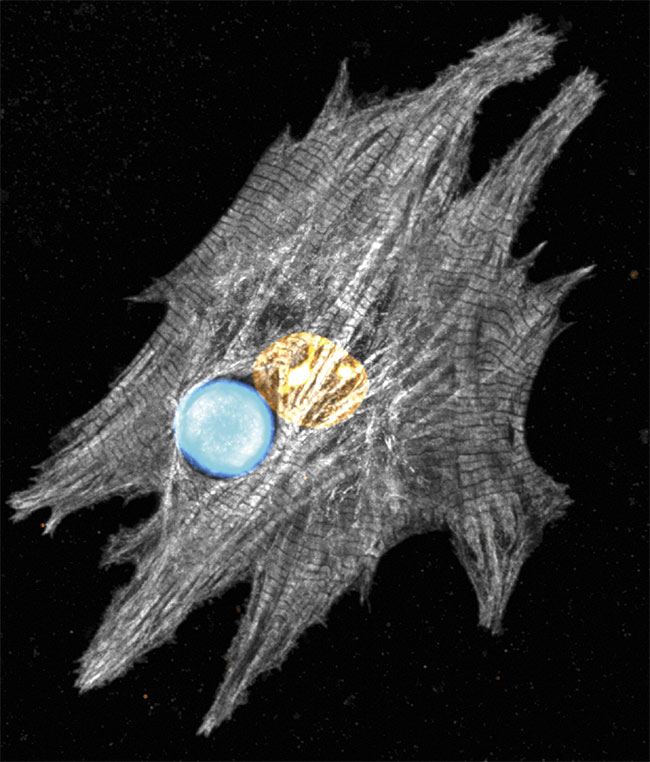
Confocal microscopy shows a microscopic laser that reveals the spectral details of a stained neonatal cardiomyocyte. Courtesy of the University of Cologne.
The team used this approach in zebrafish embryos and in thick living mouse cardiac slices, said Marcel Schubert, a professor for biointegrated photonics. In the researchers’ experiments, 15-µm spherical microlasers were placed in contact with the contractile fibrils of the zebra-fish heart. According to Schubert, each laser pulse captured a fast snapshot of the cell in the contractility cycle. Performing hundreds of these measurements per second allowed for the reconstruction of a contraction curve.
“We are measuring very small shifts in the microlaser emission wavelength, which we analyze with an optical model to extract physical information about the lasers and about the cell,” Schubert said.
The team is excited about what the future of their research will uncover, he said.
“There are different directions we would like to follow. One is a more fundamental look into the contractile properties of individual cells, which we can measure localized with very high precision. The other is the further application of WGM lasers in large and optically thick cardiac tissue and even entire organs,” Schubert said.