By filtering LED light through a narrow slit and a transmission grating, micron-width lines of tissue are illuminated by distinctive wavelengths, establishing coordinates for cellular imaging.
Nachiket Kulkarni and Dongkyun Kang, University of Arizona
Reflectance confocal microscopy (RCM) is an optical imaging technology that captures reflected light from tissue to visualize the tissue’s cellular morphologic details. The method does not require excision of the tissue or the use of fluorescent dye for differentiating details. Noninvasive imaging of human tissue using RCM was first demonstrated in the 1990s1, and over the last three decades it has been evaluated for its ability to image various types of human tissue, including skin, cornea, and oral mucosa.
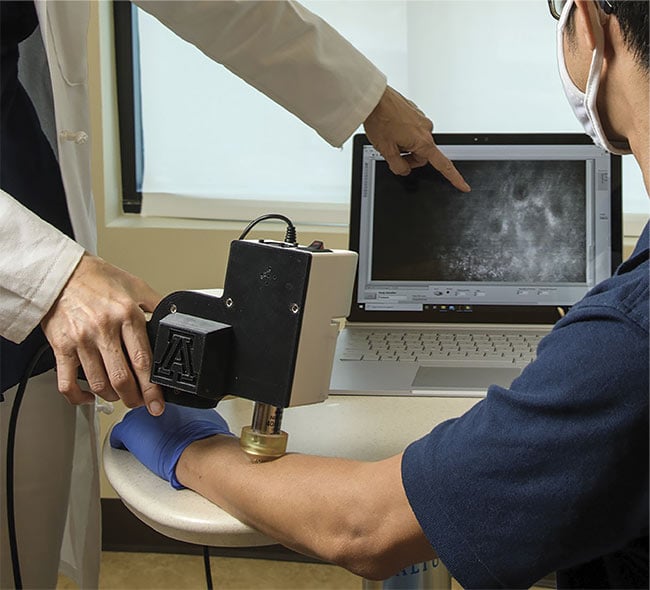
Portable reflectance confocal microscopy (RCM) can aid in the noninvasive diagnosis of various human diseases in both low- and high-resource health care
settings. Courtesy of Kris Hanning/University of Arizona Health Sciences.
Commercial equipment utilizing the advantages of RCM has also been developed for skin and eye imaging. For skin, several studies have shown that the technique provides high diagnostic sensitivity and specificity for the detection of common skin diseases2. It could be especially beneficial for diagnosing disease in low-resource settings. To diagnose some cancers, for example, RCM could image suspicious lesions noninvasively and in real time, eliminating the need for biopsy-based histopathology, which requires laboratory analysis. Adopting RCM in low-resource settings has been challenging, however, because the equipment is expensive and nonportable, and it requires training for image interpretation.
Portable RCM
In standard RCM (Figure 1), laser light is focused on a micron-size spot on a tissue of interest. The spot is rapidly scanned by two high-speed beam scanners. Light reflected back from the tissue is filtered through a detection pinhole and serially acquired by a photodetector and a high-speed data acquisition unit. This design uses high-cost, high-speed mechanical and optoelectrical components.

Figure 1. The working principles of standard reflectance confocal microscopy (RCM) and portable RCM. Top row adapted with permission from Reference 3.
With portable RCM (Figure 1), light from an LED is filtered through a narrow slit and focused on a micron-width line on the tissue3. Before being focused on the tissue, the line beam passes through a transmission grating, where different wavelengths are diffracted by different angles. This results in each line of the tissue being illuminated by a distinctive wavelength. In other words, there is a one-to-one correlation between the wavelength and the spatial coordinate of the tissue. Light reflected back from the tissue passes through the same transmission grating and is focused into a line, regardless of the wavelength. The light is further filtered by a detection slit and diffracted by another grating, which directly generates two-dimensional confocal images on a CMOS sensor.
This portable design uses low-cost, high-performance optoelectrical components, such as inexpensive LEDs and CMOS sensors, that were originally developed for consumer mobile devices. Portable RCM devices provide three benefits: They cost significantly less than standard RCM instruments, they can be made compact and portable, and their imaging speed can be increased.
Portability and low cost
The first portable RCM device was integrated with a smartphone. An LED with a center wavelength of 595 nm was used as a visible light source, and the smartphone was used for acquiring, displaying, and storing confocal images (Figure 2)4. Although the device showed promise in examining certain cellular details, it had two major limitations: slow imaging speed (4.3 fps) and limited imaging depth due to the use of visible light.
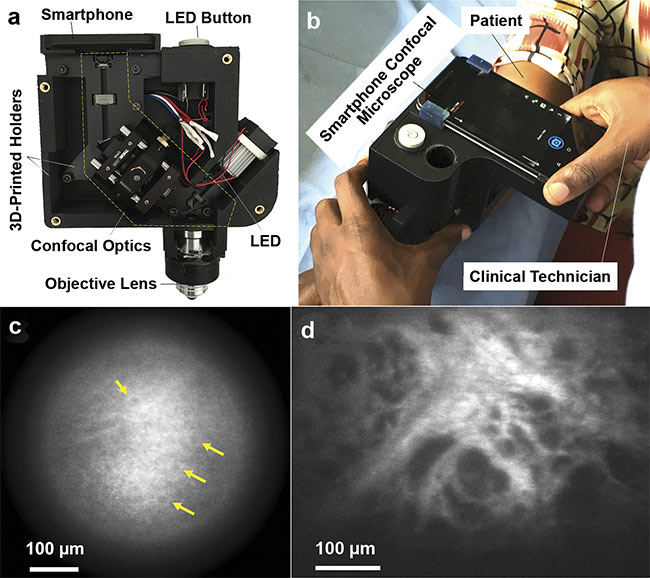
Figure 2. Photos of the first portable, smartphone-based RCM device (top row) and the confocal images obtained using it (bottom row). The RCM module is fabricated with 3D-printed holders and mounted on a smartphone (a). The instrument was tested in Uganda, where clinical technicians used it to image skin lesions (b). In vivo confocal images visualized epidermal cells (c), and ex vivo confocal images visualized irregularly shaped and sized dark spaces, which may be associated with capillaries that are indicative of Kaposi’s sarcoma (d). Adapted with permission from Reference 4.
A portable RCM device that uses near-infrared light was developed to overcome these limitations (Figure 3)5. In this device, a near-infrared LED with a center wavelength of 820 nm was used as the light source, and a monochromatic CMOS sensor was used to acquire confocal images. The image data was transferred to a laptop via a standard USB cable. The material cost of the portable RCM instrument was $5000. Its imaging speed of 20 fps enabled near real-time imaging.
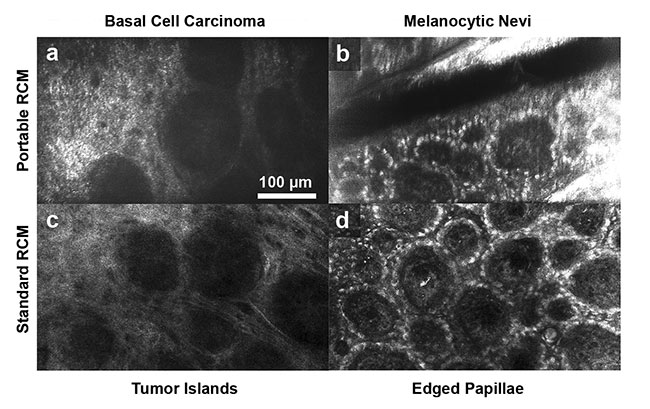
Figure 3. Confocal images of common skin lesions obtained using a portable RCM device that uses near-infrared light (top row) and a standard RCM device (bottom row). The portable device was able to similarly visualize characteristic cellular morphologic features of lesions as compared to the standard RCM device. Tumor islands in basal cell carcinoma (a, c) and edged papillae in melanocytic nevi (b, d). Adapted with permission from Reference 5.
In the portable RCM approach, the imaging speed is not limited by the speed of the mechanical beam scanners. The imaging speed of standard RCM instruments, however, is limited to 8 to 30 fps. In portable RCM, the imaging speed is mainly determined by the speed of the CMOS sensor and the signal-to-noise ratio. In preliminary experiments, the imaging speed of the CMOS sensor was increased to 180 fps (Figure 4), and a deep neural network called context-aware image restoration (CARE) was trained to reduce image noise6. Such high imaging speed could be beneficial for acquiring 3D confocal image data in real time, as well as for studying dynamic events such as blood flow.

Figure 4. Portable RCM images obtained at the high imaging speed of 180 fps. In the raw image (a), the presence of banding noise and salt-and-pepper noise poses challenges in discerning cellular details. In the deep neural network (DNN)-denoised image (b), noise strength is significantly reduced, making it almost as easy to recognize cellular features as in the ground truth image (c). Adapted with permission from Reference 6.
Although much of RCM research to date has been focused on imaging human organs that are easy to access, such as skin and cornea, the technique has also been shown to be helpful in diagnosing diseases of internal organs, such as the cervix7. To image internal organs, a portable RCM endoscope was developed. The endoscope uses a chromatic focal shift to image multiple depths of the tissue without using scanning devices8. A custom miniature objective lens with a 7-mm diameter, an 0.68 numerical aperture, and a large chromatic focal shift was developed using high-dispersion glasses. The objective lens focused various wavelengths into different depths of tissue. The bandwidth of 200 nm was mapped into the tissue depth range of 110 µm (Figure 5). The endoscope is currently being tested in Uganda to diagnose cervical pre-cancers.
Miniaturization in endoscopy
Portable RCM devices have shown promise in imaging cellular details of human tissues, and their low cost and portability could increase clinical adoption. However, several challenges to their widespread use remain.
The portable method uses a slit aperture instead of a pinhole aperture. Confocal microscopes that use a slit aperture have a shallower imaging depth than those that use a pinhole aperture, due to the slit aperture’s less effective rejection of out-of-focus light. Although portable RCM has been shown to be capable of imaging cellular details in the superficial regions of tissue, its capability to image deeper regions of tissue needs to be studied further.
The portable prototypes that have been developed for imaging skin would need to be miniaturized further to make them versatile enough for use in all clinical settings. The current prototypes can be operated using only one hand, but due to their heavy weight, extended use can be challenging, and the instruments have difficulty imaging some anatomically hidden skin lesions, such as those in armpits. Additionally, the prototypes contain an off-the-shelf objective lens that increases the device’s cost, size, and weight. A custom-made, miniaturized objective lens can be developed — as it was for the portable RCM endoscope (Figure 5) — and other optical components can be further miniaturized using the manufacturing approaches employed in the production of consumer mobile devices. A goal of future development is to develop a pen-size RCM device that can be carried in a clinician’s pocket.
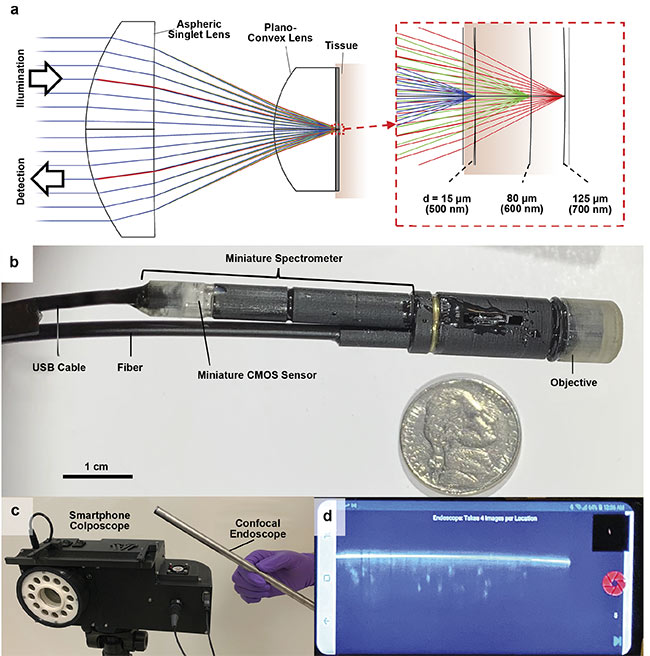
Figure 5. The working principle (top row) and photos (bottom rows) of the portable RCM endoscope. A custom miniature objective lens was used in the
endoscope, which generated a large chromatic focal shift to image the tissue cross section over a depth range of 110 µm (a). The endoscope’s diameter is
9.5 mm, and the confocal image data obtained from its miniature spectrometer was transferred over a USB cable (b). A smartphone colposcope was used in conjunction with the endoscope (c). The smartphone acquired and displayed confocal images (d). Adapted with permission from Reference 8.
Although portable RCM addresses two of the challenges of current devices — high cost and lack of portability — clinicians still need to be trained for image interpretation. AI offers great potential for analyzing RCM image data. Recently, a deep learning-based automated image segmentation method called the multiscale encoder-decoder network (MED-Net) was used to label RCM images of skin lesions exhibiting distinct morphological patterns with high specificity9. Assessment of such images could improve clinicians’ ability to make accurate and reliable screening decisions about which lesions to biopsy.
In another example of AI-based automated image analysis, an attention-based deep network automatically identified image artifacts and evaluated images quality in real time10. The use of this method could help to ensure that high-quality RCM images are acquired regardless of the experience level of the operator of the device.
By implementing simplified instrumentation along with the enhanced analysis provided by the associated software that is linked to the system, portable reflectance confocal microscopy devices could be used by less experienced health care workers in both low- and high-resource settings to provide accurate diagnoses.
Meet the authors
Nachiket Kulkarni, Ph.D., is an optical engineer at KLA Corp. He received an undergraduate degree in mechanical engineering from The National Institute of Engineering in India and a doctorate from The University of Arizona Wyant College of Optical Sciences under the guidance of Dongkyun Kang. For his doctoral thesis, Kulkarni developed a low-cost confocal endomicroscope to diagnose cervical pre-cancer; email: [email protected].
Dongkyun Kang, Ph.D., is an assistant professor of optical sciences and biomedical engineering at The University of Arizona Wyant College of Optical Sciences. His research focuses on low-cost optical microscopy devices and their clinical evaluation for use in low-resource settings; email: [email protected].
Acknowledgments
The portable RCM research has been supported by the National Institutes of Health (R21TW010221, R21TW011222, R01EY033349, R41CA261214). Nachiket Kulkarni and Dongkyun Kang are the inventors of a patent application related to the portable RCM technology presented in this article. The University of Arizona has a technology license agreement with ArgosMD Inc. involving the portable RCM technology. The authors have been granted the rights to receive royalties as a result of this licensing agreement. Kang serves as a scientific adviser to ArgosMD.
References
1. M. Rajadhyaksha et al. (1995). In vivo confocal scanning laser microscopy of human skin: melanin provides strong contrast. J Invest Dermatol, Vol. 104, No. 6, pp. 946-952.
2. M. Rajadhyaksha et al. (2017). Reflectance confocal microscopy of skin in vivo: from bench to bedside. Lasers Surg Med, Vol. 49, No. 1, pp. 7-19.
3. E.E. Freeman et al. (2018). Smartphone confocal microscopy for imaging cellular structures in human skin in vivo. Biomed Opt Express, Vol. 9, No. 4, pp. 1906-1915.
4. E.E. Freeman et al. (2020). Feasibility and implementation of portable confocal microscopy for point-of-care diagnosis of cutaneous lesions in a low-resource setting. J Am Acad Dermatol, Vol. 84, No. 2,
pp. 499-502.
5. C. Curiel-Lewandrowski et al. (2021). Preliminary imaging of skin lesions with near-infrared, portable, confocal microscopy. J Am Acad Dermatol, Vol. 85, No. 6, pp. 1624-1625.
6. J. Zhao et al. (2021). Deep learning-based denoising in high-speed portable reflectance confocal microscopy. Lasers Surg Med,
Vol. 53, No. 6, pp. 880-891.
7. K.B. Sung et al. (2003). Fiber optic confocal reflectance microscopy: a new real-time technique to view nuclear morphology in cervical squamous epithelium in vivo. Opt Express, Vol. 11, No. 24, pp. 3171-3181.
8. N. Kulkarni et al. (2021). Low-cost, chromatic confocal endomicroscope for cellular imaging in vivo. Biomed Opt Express,
Vol. 12, No. 9, pp. 5629-5643.
9. K. Kose et al. (2021). Segmentation of cellular patterns in confocal images of melanocytic lesions in vivo via a multiscale encoder-decoder network (MED-Net).
Med Image Anal, Vol. 67, p. 101841.
10. M. Wodzinski et al. (2020). Automatic quality assessment of reflectance confocal microscopy mosaics using attention-based deep neural network. 42nd Annual International Conference of the IEEE Engineering in Medicine & Biology Society (EMBC 2020).