Plasmonic Nanotweezers Keep Cool
CAMBRIDGE, Mass., Sept. 28, 2011 — A new instrument dubbed a plasmonic nanotweezer creates strong forces more efficiently than traditional optical tweezers and eliminates a problem that caused earlier setups to overheat. It may make it easier to isolate and study tiny particles such as viruses.
“We can get beyond the limitations of conventional optical tweezers, exerting a larger force on a nanoparticle for the same laser power,” said principal investigator Ken Crozier, associate professor of electrical engineering at Harvard University School of Engineering and Applied Sciences (SEAS). “Until now, overheating has been a major problem with tweezers based on surface plasmons. What we’ve shown is that you can get beyond that limitation by building a plasmonic nanotweezer with an integrated heat sink.”
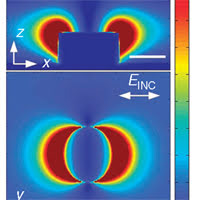
Visualization of the calculated electric field intensity around a gold pillar in Ken Crozier’s plasmonic nanotweezers. The top image is a side view; the bottom image is a view from above. Scale bar is 200 nm. (Images: Ken Crozier)
Optical tweezers have been an essential tool in biophysics for decades, often used for studying cellular components such as molecular motors. Researchers can trap and manipulate the proteins that whip a flagellum, for example, and measure the force of its swimming motion.
To create conventional optical tweezers, which were invented at Bell Labs in the 1980s, scientists shine a laser through a microscope lens, which focuses it into a very tight spot. The light, which is made up of electromagnetic waves, creates a gradient force at that focused spot that can attract a tiny particle and hold it within the beam for a short period — until random motion, radiation pressure or other forces knock it out.
The trouble with these optical tweezers is that a lens cannot focus the beam any smaller than half the wavelength of the light. If the targeted particle is much smaller than the focal spot, the trapping will be imprecise.
At the same time, the focal size places an upper limit on the gradient force that can be generated. A stronger force is necessary for trapping nanoscale particles, relative to larger, microscopic particles, so conventional optical tweezers must use a very high power laser to trap the tiniest targets.
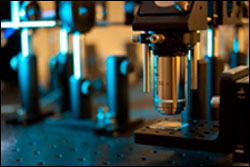
The optical table in the Crozier lab at Harvard SEAS.
To overcome these problems, researchers discovered a few years ago that they could enhance the trapping field by focusing the laser onto an array of nanoscale gold disks. The light excites the electrons at the surface of the metal, creating rapid waves of electromagnetic charge called plasma oscillations and resulting in “hot spots” of enhanced fields around the edges of the disk.
In other researchers’ designs, the tiny gold disks were arrayed on a sheet of glass, and the whole setup was submerged in water with the target particles. In tests with those devices, one problem was that the brightest hot spots were at the base of the pillars, partially inside the glass, where the particles could never be trapped. A bigger problem, as Crozier’s team discovered, was that unless they kept the laser power very low, the water boiled.
The Harvard team solved both problems by replacing the glass with a piece of silicon coated in copper and then gold, with raised gold pillars. These materials are much more thermally conductive than glass, so they act as a heat sink.
“The gold, copper and silicon under the pillars act just like the heat sink attached to the chip in your PC, drawing the heat away,” said lead author Kai Wang, who completed the work at SEAS and is now a postdoctoral fellow at Howard Hughes Medical Institute.
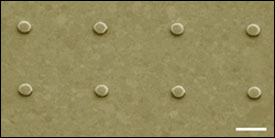
A false-color SEM image of the gold nanopillars. Underneath the flat gold surface is a layer of copper, and both metals have been evaporated onto a sheet of silicon. The top surface, with the pillars, is created through a process called template stripping, which makes it very smooth. Scale bar is 1 µm.
The new device reduces the water heating by about a hundredfold and produces hot spots at the top edges of the pillars, where the team trapped polystyrene balls as small as 110 nm.
In an unusual twist, the researchers discovered that they could rotate the trapped particles around the pillars by rotating the linear polarizer on the optical table where they conducted the experiments. Going further, they replaced the linear polarizer with a circular one and found that the particle automatically and continuously traveled around the pillar.
As the electromagnetic field circled the pillar, it created an optical force that pushed the particle. Despite the fact that the electromagnetic field traveled at about 1014 rotations per second, the balance between the optical force and the fluid drag resulted in a particle velocity of about 5 rotations per second, effectively a terminal velocity.
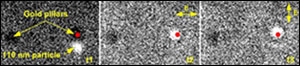
Particle trapping and rotation in action.
“This phenomenon seems to be entirely novel,” Crozier said. “People have trapped particles before, but they’ve never done anything like that.”
As tools for trapping and manipulating nanoparticles become more advanced, the potential applications in biophysics are extensive. One remaining challenge, however, is the researcher’s ability to detect and quantify the motion of such tiny particles.
“It’s going to be harder and harder to precisely track the center of the particle when we do these manipulations,” Crozier said. “Progress in the realm of sensing tools will need to keep up.”
The research appears in Nature Communications.
For more information, visit: www.harvard.edu
Published: September 2011