Optics-based instruments will help crack the mysteries of the cosmos
What would we know about the universe without
the aid of optics? A whole lot less than we do now, to be sure. Optics has always
played a significant role in both manned and unmanned space missions, while astronomical
study would be largely inconceivable without the telescope and the myriad imaging
modalities introduced in the four centuries since Galileo.
As much as optics-based instruments have told us already, though,
continued development of the technology is enabling us to look farther and probe
deeper than ever before.
The Mars missions offer a good example of how optics and photonics
can help to reveal yet more about the universe. “Curiosity,” the next
Mars rover, will seek to determine whether the environment on the red planet once
was – or might still be – able to support microbial life. Due to launch
in November 2011, the rover will carry 10 instrument packages, including ChemCam,
which will employ laser-induced breakdown spectroscopy (LIBS) to measure the chemical
content of rocks at distances of up to 22 feet.
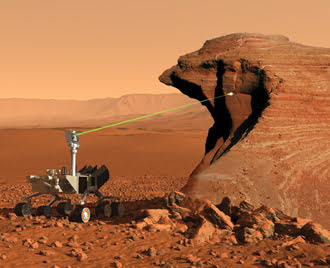
The next Mars rover will carry a number of instruments to aid in the search for habitability
on the planet. Among these will be ChemCam, which will use laser-induced breakdown
spectroscopy to study the preservation of carbon-bearing materials. Courtesy of
Jean-Luc Lacour, CEA, and Los Alamos National Laboratory.
This latter capability is essential. One of the reasons ChemCam
was selected for the mission, said Roger Wiens of Los Alamos National Laboratory
in New Mexico, the instrument’s principal investigator, was that planetary
exploration with purely passive methods has proved something of a challenge. One
example: In 2004, “Opportunity,” the second of two rovers in the Mars
Exploration Rover (MER) mission, landed directly in a small crater, much to the
delight of mission specialists. Taking full advantage of this bit of good fortune
proved difficult, though.
“When the rover’s cameras were deployed,” Wiens
said, “lo and behold, they saw the very first extraterrestrial rock outcrop
right before them. It turns out it was sedimentary rock, and yet the remote sensing
really couldn’t tell it was sedimentary. That kind of stoked the desire to
have an active technique that would be able to brush away dust, so to speak, without
actually touching the rock.”
ChemCam will allow NASA to do just that. By firing laser pulses,
it can penetrate dust layers on the rock and even obtain depth profiles to determine
whether there are chemical weathering layers – which can reveal quite a bit
about weather over very long periods on Mars, Wiens said. And of course, clearing
away the dust also enables study of the pristine rock underneath.
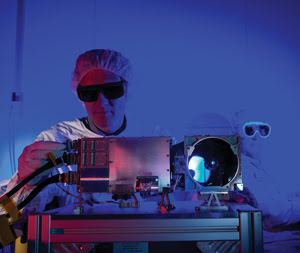
ChemCam can determine the elements present in a Mars rock by observing
the light-emitting plasma from material ablated from the rock and by matching the
spectral emissions against a library of known chemical compounds. Courtesy of Los
Alamos National Laboratory.
Here’s how it works: ChemCam’s laser, imager and telescope
reside in the instrument’s mast unit, mounted on the rover’s remote-sensing
mast 2.01 m above the ground. Brief, 5-ns pulses from the 30-mJ Nd:KGW laser operating
at 1067 nm produce light-emitting plasmas from material ablated from the rock. The
telescope observes the light and delivers it to three spectrometers in ChemCam’s
body unit, nestled inside the body of the rover. The spectrometers, with a combined
spectral range of 240 to 860 nm, have resolutions between 0.15 and 0.65 nm full
width half-maximum. The spectral emissions can be matched against a library of known
chemical compounds; thus, scientists can determine the elements present in the sample.
LIBS analysis generally consists of 50 laser pulses at 3 Hz, the
first several of which will be for cleaning. The spectra from the remaining pulses
typically are averaged together to achieve better statistics. In some cases, however,
as in weathering rind analyses, they can be analyzed separately.
Although the LIBS technique and the technology used in ChemCam
have been established, the researchers found that, off the shelf, they weren’t
always suitable for this application. As a result, Wiens said, they had to revise
the design several times while developing the instrument. One example: The original
design included three optical fibers connecting the telescope to each of the spectrometers.
Images in the LIBS literature showed larger plasmas measuring more than a centimeter
wide at Mars pressures, which should have allowed the light to be captured across
several fibers at the focal point of the telescope.
However, when the team members did their homework and checked
the spatial distribution of the plasma light, they found that the portion of the
bright plasma yielding strong emission line signals was much smaller – less
than a millimeter in diameter. So they redesigned the instrument to use a single
optical fiber connecting the telescope on the mast to the spectrometers in the body.
The team also found that it had to replace the original spectrometer
CCDs – very simple and inexpensive commercial CCDs – with more complex
devices, as the simpler ones failed a radiation test.
Using these various optical technologies, ChemCam will assist
in the search for habitability on Mars. The MER rovers and orbital spacecraft of
the past decade found abundant evidence for water in Mars’ past, fulfilling
NASA’s mantra to “follow the water,” Wiens said. The Curiosity
rover takes Mars exploration to the next level by studying the preservation of carbon-bearing
materials.
The landing site selection is focused on concentrations of clay
minerals and features such as river deltas. The rover is equipped with sensitive
mass spectrometers and a gas chromatograph for detecting and identifying carbon-bearing
compounds, a critical elemental capability lacking in previous rovers. ChemCam will
also aid in the habitability search by remotely detecting carbon, hydrogen and other
elements important to the question of habitability.
The ChemCam instrument suite was a joint development between France
and the US, with the mast unit contributed by CNES, the French Space Agency, and
the body unit developed and built at Los Alamos National Laboratory, which led the
project. The laser was developed and built by Thales. The spectrometer optical design
was provided by Ocean Optics. Jet Propulsion Laboratory, which is building the Curiosity
rover, and Goddard Space Flight Center also contributed optical expertise for the
ChemCam instrument.
Peering across space and time
The next several years will see the launch of other important
optics-based instruments, such as those developed for the James Webb Space Telescope,
or JWST – the successor to the Hubble. The JWST will consist of three major
elements: the Spacecraft Element; the Optical Telescope Element, which will gather
the light from afar and deliver it to the instruments; and the Integrated Science
Instrument Module, with the instruments themselves.
These will include the Mid-Infrared Instrument, or MIRI, which
was designed to aid scientists in a number of areas: studies of the first light
in the early universe and of the formation of planets around distant stars, among
others. Such events are often hidden from ground-based telescopes and even the Hubble
by clouds of dust surrounding newly formed stars, for example. With MIRI, though,
scientists will be able to peer through the dust; the instrument will be sensitive
to light in the range of 5 to 28.3 µm, which can penetrate the clouds.
“It will be the most sensitive space- or ground-based telescope
– the most sensitive astronomical instrument – working in that waveband
by several orders of magnitude,” said John Pye, Space Research Centre manager
at the University of Leicester and the university’s lead staff member for
MIRI.
The instrument will consist of two main modules: an imager and
a medium-resolution spectrograph. The imager will offer broad- and narrowband imaging,
phase-mask coronagraphy, Lyot coronagraphy and prism low-resolution split spectroscopy
in the 5- to 10-μm range, using a 1024 x 1024-pixel arsenic-doped silicon (Si:As)
sensor chip assembly. The medium-resolution spectrograph will record spectral and
spatial data, using four integral field units – here providing four simultaneous
fields of view. This instrument will employ two 1024 x 1024-pixel Si:As sensor chip
assemblies.
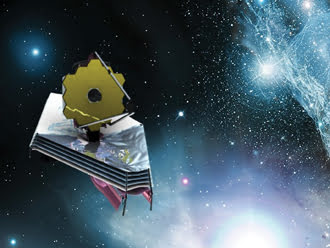
The James Webb Space Telescope – an artist’s impression of which is shown here – will carry, among other devices, a Mid-Infrared Instrument (MIRI) that will advance understanding
of, for example, the first light in the early universe and the formation of planets
around distant stars. Courtesy of the European Space Agency.
The greatest challenge in developing MIRI, Pye said, was ensuring
operation at temperatures within 7° of absolute zero. The James Webb Telescope
ultimately will make its home at L2, a gravitational pivot point some 150,000 km
from Earth – moving away from the sun. Here, shaded from the light of both
the sun and the Earth, it is cool enough for the instrument to record in the mid-infrared,
and thus to obtain the measurements that will help scientists understand a range
of phenomena.
“Although the instrument was assembled at room temperature,”
Pye said, “we had to make sure it maintains alignment as it cools down.”
They achieved this by using aluminum as much as possible in the design of the instrument,
including most of the optical components. The filters are not aluminum. The material
is light, strong and relatively low-cost, Pye said, and at the appropriate grade
has extensive “space heritage.”
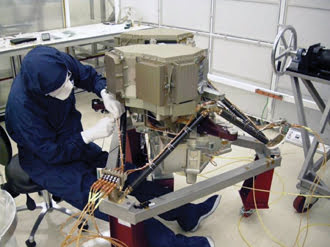
MIRI is currently undergoing testing at
Rutherford Appleton Laboratory in the UK. Once completed, the instrument will be
shipped to NASA’s Goddard Space Flight Center in Maryland, where it will be
integrated with the James Webb Telescope’s Integrated Science Instrument Module.
Courtesy of MIRI European Consortium, Rutherford Appleton Laboratory.
MIRI, which is being developed by NASA and a consortium of European
partners allied with the European Space Agency, is being prepared for eventual deployment.
The MIRI team is led jointly by professor Gillian Wright of the Science & Technology
Facilities Council (STFC) Astronomy Technology Centre in Edinburgh, UK, and professor
George Rieke of the University of Arizona, Tucson. The subassemblies – including
the imager, the spectrometer optics, and the input-optics and calibration module
– have been integrated into a flight model of MIRI, which is currently undergoing
testing at STFC’s Rutherford Appleton Laboratory in the UK, using a chamber
specially designed to reproduce the environment at L2.
Once the tests are completed, MIRI will travel to NASA’s
Goddard Space Flight Center in Greenbelt, Md., where it will be integrated with
the James Webb Telescope’s Integrated Science Instrument Module. Launch is
planned for no sooner than 2014.