CRISTINA KURACHI, SÃO CARLOS INSTITUTE OF PHYSICS, UNIVERSITY OF SÃO PAULO
Photodynamic therapy is a powerful technique in the local, selective treatment of cancer and infectious diseases, but customized optical device protocols must be added to the mix for major clinical translation.
Photodynamic therapy (PDT) is a robust treatment procedure that can be designated to treat some types of cancer, as well as infectious diseases and other tissue disorders. It involves the use of a photosensitizer — a chemical compound that is activated by light — which results in the production of reactive species (mainly singlet oxygen) and further induction of cell death.
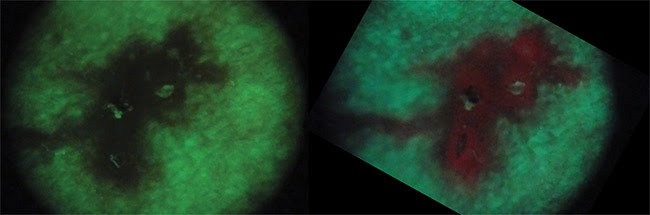
Courtesy of Cristina Kurachi.
Because of its action mechanism, PDT shows only a local response, and the target tissue must be completely irradiated for the therapy to succeed. There must also be minimal concentrations of the photosensitizer and oxygen to produce an effective PDT response. Now, biological features and challenges are involved to achieve and improve the PDT clinical translation.
Photodynamics
As a local treatment modality that uses light at a specific wavelength band to activate a photosensitizing molecule (photosensitizer), PDT mainly transfers energy to oxygen, generating reactive oxygen species (ROS) that will induce cell death.
Photodynamic action has been used to treat cancer lesions, infected lesions, and other tissue disorders such as age-related macular degeneration, diabetic foot ulcers, and psoriasis. Figure 1 shows a sequence of four frames from a video, taken by a fluorescence microscope, of amoeba cells treated with curcumin. Initially, the amoeba cells appear as dark round cells, with low native
PDT is a powerful technique and several protocols
have been proposed with efficient response, but a major clinical translation will take place only when customized optical device protocols have been designed.
fluorescence under 450-nm excitation (Figure 1a).
Later, curcumin molecules are internalized and the cells begin to show the intense green fluorescence characteristic of curcumin (Figures 1b and 1c). Finally, the same excitation light that induces the fluorescence also produces the photodynamic reaction. The membranes are one of the main cell targets of the PDT action, which results in their destruction and the release of the intracellular materials (Figure 1d).
For a successful treatment, the target tissue must be well-oxygenated, and enough concentration of photosensitizer within the cells and uniform irradiation above the threshold energy dose must be achieved. This threshold dose is the minimal amount of light energy, defined by cell in vitro and animal in vivo model experiments, usually stated in J.cm-2. All three of these components — photosensitizer, oxygen, and light — must be present in high enough quantities to obtain overall cell death.
As shown in Figure 1, it is not so complicated to achieve efficient PDT parameters in planktonic cells; for clinical PDT, this is not the case. Mammalian cells are organized in tissues, and even microorganisms that infect lesions are mostly present in biofilm, a well-structured community. Photosensitizer distribution within tumors located in distinct organs and patients will present individual variations on the pharmacokinetics for systemic administration.
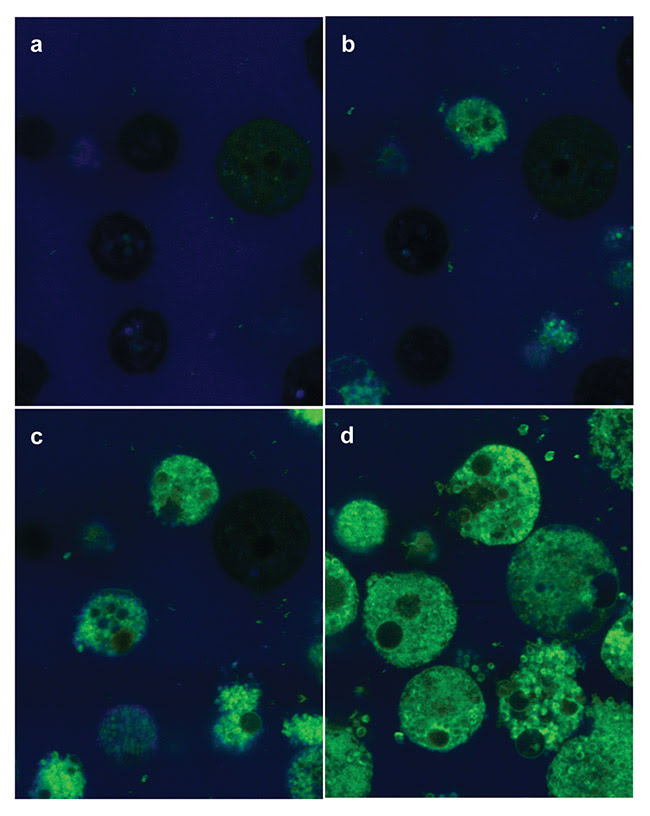
Figure 1. Amoeba cells incubated with curcumin and imaged with fluorescence microscopy under 450-nm excitation. The amoeba cells show a low native fluorescence (a), but when the curcumin is internalized, an intense green fluorescence can be seen (b and c). When the sample continues to be illuminated, the photodynamic reaction takes place. Main cell damage at the membranes results in cell death, mostly by cell membrane breakdown (d). Courtesy of Cristina Kurachi.
The PDT element that depends only on the native tissue characteristics, mainly the vascularization network and supply, is the oxygen present within the cell/tissue environment. Even though the concentration and distribution of the other elements — photosensitizer and light — are also dependent on tissue properties, they may be improved when using customized PDT strategies.
Treatment strategies
The porphyrin-derivative compounds systemically administered via intravenous injection are the first generation of PDT photosensitizers. In their case, the photosensitizer delivery to the tumor mostly depends on the vascular network; the more vascularized the tumor, the higher the photosensitizer supply. Varying the time interval between the photosensitizer injection and tumor irradiation results in a higher vascular (shorter interval) or cellular (longer interval) effect.
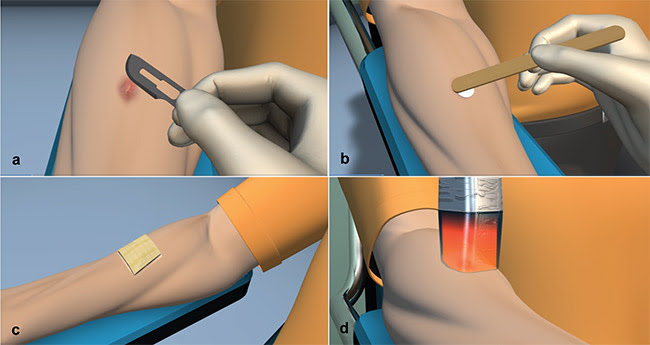
Figure 2. Sequence of photodynamic therapy (PDT) protocol for basal cell carcinoma (BCC): superficial curettage using a scalpel blade and cleansing of the lesion with a transparent cleaning solution (a); topical application of 20 percent methyl-aminolevulinate (MAL) cream over the lesion, including a 2-mm margin of normal skin (b); curative avoiding of light exposure for three hours (c); and lesion irradiation at 630 nm (d). These clinical protocols are repeated one week later. Courtesy of Cristina Kurachi.
Higher concentration of malignant cells in comparison to normal cells has been described, constituting one of the mechanisms of PDT selective response. To improve this selectivity, several groups of researchers have been working on designing intelligent molecules that can target specific cells, as well as on more efficient drug delivery — for example, a method that is nanoassembly-based.
The main indication of topical PDT is presently basal cell carcinoma (BCC), whereby a prodrug is locally administered over the lesion to induce the production of an endogenous photosensitizer, protoporphyrin IX (PpIX). Conventionally, either aminolevulinic acid (ALA) or methyl-aminolevulinate (MAL) is administered in a cream and incubated for three hours before lesion irradiation (Figure 2). This time interval is recommended to ensure that enough ALA molecules are internalized at the epidermal cells and converted to PpIX. Figure 3 shows an example of a superficial BCC treated by two MAL-PDT sessions in a one-week interval.
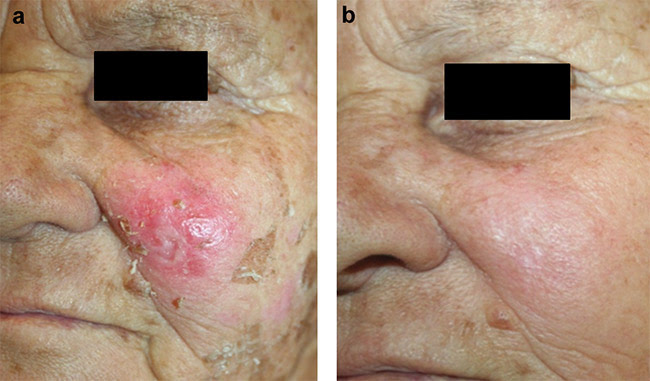
Figure 3. Example of a superficial basal cell carcinoma (BCC) at the left cheek (a) and the clinical aspect presented 30 days after two sessions of photodynamic therapy (PDT), with 20 percent methyl-aminolevulinate (MAL) cream and irradiation at 630 nm and 150 J/cm2. Courtesy of Dr. Ana Gabriela Salvio/Amaral Carvalho Hospital.
Unfortunately, ALA distribution and further PpIX production does not occur uniformly within the tumor, mostly because of the inherent heterogeneities that affect the transdermal drug delivery. This PpIX distribution, when it is highly unequal and not properly solved, will affect the overall PDT response. Wide-field fluorescence detection is a simple and clinically feasible technique to evaluate this condition. The clinician can easily perform a noninvasive qualitative evaluation of the PpIX production at the lesion surface. Since the native skin fluorescence is at the green spectral region, the BCC shows a loss of fluorescence and the PpIX shows a red fluorescence emission (Figure 4).
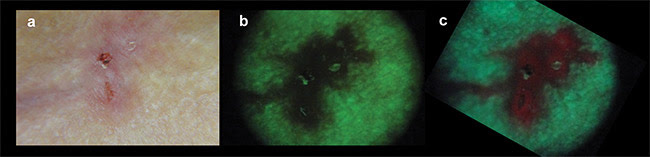
Figure 4. Clinical appearance of a basal cell carcinoma (BCC) under white light illumination (a). Wide-field fluorescence imaging under 400-nm excitation, showing the loss of autofluorescence at the lesion when compared to the surrounding normal skin (b), and the characteristic red PpIX fluorescence after three hours of incubation of 20 percent methyl-aminolevulinate (MAL) cream (c). Courtesy of Dr. Ana Gabriela Salvio/Amaral Carvalho Hospital.
Different strategies have been proposed to improve transdermal ALA delivery. One of these is the use of a high-power CO2 laser to produce a microhole grating. This is followed by an application of the ALA cream, assisted by ultrasound to enhance the cream’s penetration into the holes. Another approach is the use of a soluble microneedle patch, in which the ALA is continuously delivered until the material is completely dissolved inside the lesion. More recently, ALA delivery using a dermographic device has been investigated. These three strategies have shown promising results thus far, after improving the PpIX production in quantity and homogeneity.
Lesion irradiation is another PDT treatment element that can be improved. As previously mentioned, to produce cell death a threshold dose must be achieved at the tumor site or in the whole biofilm. A main limitation in this area relates to the visible light attenuation of biological tissues. Drug development for PDT also includes the design of photosensitizer molecules that absorb at the optical window, in the 700- to 1000-nm range.
Optical properties and target tissue anatomy are highly distinct, depending on inherent tumor properties and organ site. As a consequence, PDT irradiation planning is a challenge because it cannot be standardized for all cases. Optical coupling will vary, depending on the surface characteristics as well as on the absorption and scattering coefficients of the target tissue.
Considering surface irregularities, some researchers have indicated using optical matching gels over a tumor surface just before irradiation of the lesion. This enhances optical coupling within the tissue. Additionally, several groups of researchers have been supporting the idea of customized irradiation planning that involves the previous evaluation of a tumor’s optical properties — thus predicting light attenuation and penetration depth — and then using this information to determine irradiation parameters (irradiance and fluence). Another approach is to use interstitial fibers, not only irradiating the tumor’s surface but also irradiating the tumor from the inside out.
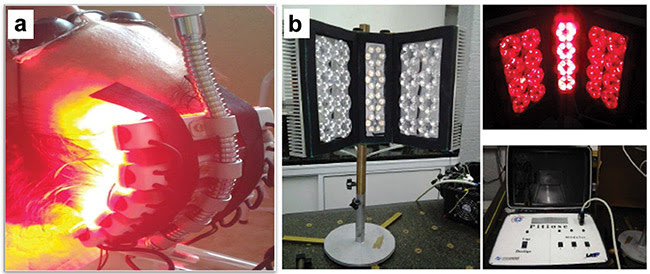
Figure 5. Two examples of LED-based devices: a prototype for actinic keratosis and field cancerization, with application at the head (a), and a prototype for horse pythiosis (b). Courtesy of Cristina Kurachi.
LED-based devices have been successfully replacing laser devices for PDT applications where the target tissue is superficial and directly accessed, such as on skin and in oral cavities. LED arrays can be assembled in different optical configurations (more easily than with lasers), from small to larger irradiation areas and geometries. Two examples are presented in Figure 5.
LED-based devices have been designed to deliver PDT treatment for skin field cancerization (Figure 5a), cervical intraepithelial neoplasia, bacterial oropharyngitis, periodontal disease, and onychomycosis, among others, and always with this concept of customizing the optical device according to the organ geometry and irradiation needs.
Figure 5b shows the development of an LED prototype that has been used to treat horse pythiosis — an infection caused by the oomycete Pythium insidiosum. Conventional treatment involves the surgical resection of the infected wound, since there is no efficient antibiotic therapy. Horses are usually infected at the feet, but also in the neck and the stomach. The LED-based device was designed to irradiate a large area, with angular freedom to better produce a uniform irradiation of the lesion; the angle is adjusted depending on the lesion site.
PDT is a local and selective treatment technique that has mostly been applied for cancer and infectious disease. Even though it is a powerful technique, and several protocols have been proposed with efficient response, a major clinical translation will take place only when customized optical device protocols have been designed. Developments in optical instrumentation, photosensitizer molecules, and delivery have been fulfilling these needs, and more clinical translation and new protocols are on the way.
Meet the author
Cristina Kurachi, Ph.D., is a professor at the University of São Paulo and a researcher at the National Council for Scientific and Technological Development (CNPq) in Brazil. She has bachelor’s and master’s degrees from the University of São Paulo, where she also received her Ph.D. She focuses on biophotonics, focusing on light interaction in biological systems, optical diagnosis, and photodynamic therapy that is applied to cancer and infected tissues.
Acknowledgments
The author would like to thank collaborators and students at the Optics and Photonics Research Center at the University of São Paulo’s São Carlos Institute of Physics. She would also like to express appreciation for the financial support provided by the Centers for Research, Innovation and Dissemination (CEPID) program of the São Paulo Research Foundation (FAPESP) and by the Ministry of Science, Technology, Innovation and Communications.