Originally characterized as a solution in search of a problem, lasers now are the answer to a host of problems. Two recent examples show just how laser optics enable new applications. One promises to make a common safety tool – the light barrier – better able to handle long runs, while the second could allow laser-powered precise microsurgery without harmful heating. Both are possible because of lasers, detectors and the application of the appropriate optics.

A light barrier (schematic shown on top, actual setup on bottom) consisting of two line lasers and a photodetector array forms a rectangular detection area. Reprinted with permission of Applied Optics.
Building a better barrier
Light barriers, as their name implies, are constructed out of light, not metal or another material. They protect people from machines – and vice versa. For example, they wall off automated machinery and shut down robots if someone crosses a threshold. In that way, machines and people can coexist with greater safety on an assembly line.
But not all light barriers are the same. They fall into two basic categories: discrete and continuous light beams. Eldar Musa, a professor of electronic engineering at Uludag University in Bursa, Turkey, noted that the two types are not equal. “Continuous beams have an advantage.”
However, discrete systems often are used, especially in cases where only a small area must be covered. In part, that’s because of their simplicity.
They have a source, such as an LED, and a detector. Each source-detector pair can be thought of as a bar in a fence. Cordoning off an area requires placing pairs along a line, with the distance between each pair setting the minimum detectable object size. In operation, the sources fire sequentially, with the opposing detector synchronized to the pulses. Doing so ensures that the detector correctly picks up the light from the appropriate source.
Although this approach works for smaller spans, it runs into problems as it is scaled up, Musa reported in the July 1, 2008, issue of Applied Optics. A longer run means that more source-detector pairs must be used, resulting in a more expensive system that breaks down more often. Also, a longer run means that cycling through the entire source-detector array takes longer. Thus, the response time of the system goes up.
The problem with scaling up appears in other dimensions as well. For example, increasing the distance between the source and the detector while maintaining the performance of the system means that the source must be brighter, the optics better or the detector more sensitive. All of these options present problems.
Musa, therefore, looked at how best to implement a barrier based on a continuous light beam. He used a line laser from Lanics Co. Ltd. of Seoul, South Korea, selecting this type because of the wide variety available. For detectors, he used a linear array of phototransistors from Osram GmbH of Munich, Germany, with the separation between detectors determining the minimum detectable object size.
Because of the setup’s geometry, a single laser yielded a triangular detection area, with the beam fanning out from a point to cover the photodetector array. However, Musa noted, the detection area can be converted into a rectangular shape with the addition of a second laser. A third laser situated midway between the other two produced an even larger detection area.
Rather than fire all the lasers continuously, he borrowed a technique from the discrete approach and used a sequential pulsed driving method. Doing so allowed him to extract the object’s location.
Although the prototype worked as expected, commercial prospects for such a system are unclear. Musa also noted that he is not done designing and building a better light barrier. “The next step is to use only one laser to form a rectangular-shaped detection area.”
Creating a cold laser instrument
George I. Zheltov, chief research officer with B.I. Stepanov Institute of Physics at the National Academy of Sciences of Belarus in Minsk, recently applied laser optics in the first step toward realizing an old dream. He has long wanted to create a precision laser-based instrument for delicate microsurgery. The trick was that the instrument would keep heating to a minimum while still allowing the removal of tissue.
“There are medical cases in which the great heating of tissues is absolutely indispensable and others where it is extremely undesirable,” Zheltov said, explaining why such an instrument would be useful. As examples, he cited neurosurgery, ophthalmology, otolaryngology and other disciplines in which microsurgeries are performed. All require the removal of diseased tissue while avoiding damage to the surrounding healthy structures such as nerves, blood vessels and membranes.
Zheltov was lead author of a July 10, 2008, Applied Optics paper that described what could be the beginning of such a precise, yet cold, laser-based instrument. Others on the team also were from the institute. The group used a custom-built Raman laser operating at 1626 nm to create bubbles in water – a cavitation process.
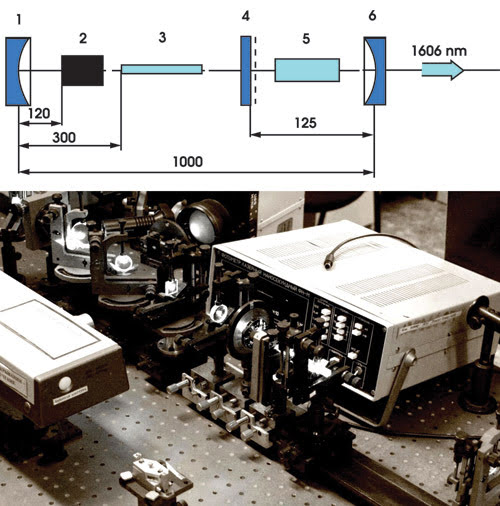
With this custom-built Raman laser (schematic on top, setup on bottom), researchers created bubbles in water that could be useful for precise microsurgery without heat damaging nearby tissue. Courtesy of George I. Zheltov, B.I. Stepanov Institute of Physics.
The mechanism behind the bubble formation is tied to the acoustic waves created in the water by nanosecond-duration laser pulses. The resulting waves have both a positive and negative phase. The latter gives rise to cavitation pockets – provided that the irradiation is above a threshold.
Trying to produce the required bubbles with a single laser pulse would make the process inefficient because it would require that everything happen on a timescale about the same as the laser pulse duration. According to theory, the bubbles can be long-lived because it takes energy to collapse them. So the researchers used a train of pulses – with each adding to the cavitation field – to build up the bubbles more slowly and efficiently.

Bubbles on demand, with size controlled by laser intensity. Shown on the top is a bubble formed precisely at the intensity threshold. At bottom is a bubble formed at approximately twice the intensity. Reprinted with permission of Applied Optics.
This approach, however, placed special demands on the system; for example, the creation of the irradiated area had to approach a spherical form. Achieving that meant that the power of the spreading acoustic wave would fall off very quickly, guaranteeing localized action.
To meet these needs, the group built a custom Raman laser, using mirrors, a barium nitrate crystal, a passive modulator and an active YAG element. In testing their concept, they irradiated water in a cell. They observed the bubbles formed by the first laser using a CCD camera by scattering light from a second low-power laser off the water. Tests showed that the bubbles formed as expected, with no cavitation when the intensity was just below the threshold. At higher intensities, stable bubbles formed, their size dependent upon the amount of energy above the threshold.
This could be the basis for a precise tool to remove tissue, Zheltov noted. “Because of the small mechanical strength of the overwhelming majority of biotissues, their cavitation damage can be observed upon laser heating to temperatures much lower than the coagulation and boiling point under normal conditions.”
The group has applied for a patent and is in the first stages of a project intended to create an instrument for fragmentation of bloodless tissues such as cartilage and hard cataracts. Lasers have some advantages in these procedures because they allow precise localized action. By employing a fiber, the technique also could be used in difficult-to-reach places. The instrument will be built after detailed experimental investigation of the approach on living tissue, Zheltov said.