Optical coherence tomography has emerged as a robust tool in the comprehensive diagnosis of medical conditions, but it needs to be more accessible and affordable for practitioners.
ADAM WAX, DUKE UNIVERSITY
Medical devices at the point of care allow clinicians to do what they do best: determine a patient’s exact condition and a course of treatment. These technologies work best when they fit seamlessly into the provider-patient workflow without a steep learning curve or worry about the underlying scientific principles, and without the high cost seen for so many medical technologies.
Optical coherence tomography (OCT) is an imaging technology that has become well-known in recent years for its proven diagnostic ability, particularly in the eye care realm. This technology is now the gold standard for diagnosing eye diseases such as diabetic retinopathy and macular degeneration. Its ability to create depth-resolved, cross-sectional images allows physicians to examine the various layers of the retina and see the earliest signs of disease. While OCT has seen wide adoption by eye care professionals, the high cost of imaging systems ($40,000 to $100,000 or more) has limited access primarily to large eye centers.
What if OCT could be more affordable, accurate, and accessible for all eye care professionals, including optometrists? What if primary care practitioners could use OCT to diagnose early eye disease? For this technology to become a true point-of-care solution, it needs to be less expensive, easier to use, and more portable.
Bench to bedside
The BIOS research group in Duke University’s Department of Biomedical Engineering has worked for many years developing biomedical imaging devices and systems. The path from laboratory to bedside can be challenging, requiring investment of significant time and funding. The end product is often a device that is costly to produce commercially, ultimately limiting how and when it can be used for patients.
But the BIOS research group recently developed a low-cost OCT system, so more providers and patients can benefit from its accurate and noninvasive imaging capabilities. The system is portable, robust, and capable of operating at the point of care.
For many patients with compromised vision, including those with advanced diabetes, traveling to a central eye care facility for an OCT exam can be difficult. This raises a barrier to receiving necessary follow-up exams. In most communities, primary eye care providers are optometrists who may not provide OCT imaging because of the cost of a system. Its high price also restricts its availability in developing countries, where the value of clinical OCT is understood yet remains cost-prohibitive.
To address the affordability issue, the BIOS research group created a prototype OCT system with a target sales price of $10,000. This system provides performance comparable to other entry-level OCT imaging systems at a fraction of the cost. By delivering OCT imaging at this price, the economics of care dramatically shift, disrupting the market by making it accessible to most point-of-care clinics.

Figure 1. A low-cost OCT system, optical engine, and scanner positioned on a microscope stand (a). Top view of the system interior (b). Adapted with permission from reference 1.
Figure 1 shows the imaging system developed by the BIOS research group1, along with an inside view and block diagram. The researchers were able to significantly reduce component costs by taking a system-level approach; that is, instead of basing the design on optimizing the performance of individual components, the system was designed to provide sufficient imaging function but allow for compromise on individual components.
Driving down costs
The main cost drivers of most spectral-domain OCT systems are the light source and spectrometer. In the low-cost design, a system-level approach achieves the desired performance. The light source is an uncooled, fiber-coupled superluminescent diode (SLD), which is lower cost but can provide ample performance. The spectrometer and software are designed to accommodate the intensity and spectral fluctuations expected for this source. The custom spectrometer design used is tolerant to misalignment and permits the use of a 3D-printed housing rather than precision-machined metal parts.
The cost of the control electronics also was reduced. A mini PC is integrated into the system, eliminating the need and cost for an external PC or laptop. All of the instrumentation in the system is interfaced and powered through the PC’s USB ports. To synchronize data acquisition with beam scanning, a separate microcontroller acts as a timing generator — it receives instructions from the PC and sends control signals to the sensor in the spectrometer and MEMS mirror, which scans the beam. The system includes a liquid lens, controlled by the software on the PC. This allows users to control the focal plane of the scanner.
Initially, the system was developed using MATLAB and LabVIEW software. However, to improve imaging speed, the group implemented a custom routine on the PC’s graphics processing unit (GPU) using OpenCL, an open standard for parallel computing.
The low-cost OCT system delivers an axial resolution of 7 μm in air, a lateral resolution of 17.6 μm, and 2.8 mm of imaging depth. The scan range was 7 × 7 mm, delivering 700 µm to the sample. The A-scan rate of 8.8 kHz produces roughly 12 B-scans per second at a signal-to-noise ratio (SNR) of nearly 100 dB. Figure 2 shows example images of biological tissues. Most significantly, the device is not only inexpensive, it also offers a small form factor. The list of materials for the prototype amounted to $7164, and it weighs only 6 lbs, including the integrated PC.
Figure 2. Images acquired with a low-cost OCT system: a pig cornea (a); lens and iris (b); iridocorneal angle (c); murine skin (d). Scale bar = 200 μm. Adapted with permission from reference 1.
Its volume of 524 in.3 is roughly the size of a shoebox. In comparison with other entry-level commercial systems, performance is comparable, but the cost and size are as much as 80 percent less1.
The low-cost OCT system has been made commercially available by Lumedica with its OQ LabScope. This is an affordable, accurate, easy-to-use OCT system that offers laboratory-grade performance. Customized versions also are available with application-specific features and capabilities. While this product is not yet approved for retinal imaging in patients, a product for the ophthalmic market is in development. The OQ Eyescope is expected to be FDA cleared and on the market by the end of 2018 to enable use of OCT at the point of care by a wide range of eye care professionals.
Dual-axis OCT
While OCT has found widespread adoption for retinal imaging, it has yet to become widely adopted for other tissues that require greater depth penetration. Market research has shown that this is partly due to the price point of most OCT systems, but also because of limitations in performance. In particular, OCT can only penetrate 1 to 2 mm in tissue such as
skin, which scatters light. However, to provide a clinically valuable skin diagnostic, this depth range must be extended to 3 to 4 mm for penetrating beyond superficial layers to the dermal junction. With this improvement, OCT could be used by dermatologists to examine suspicious moles for cancer, by surgeons to assess the health of tissue grafts, and by ER doctors to assess the depth of burns for prescribing treatment.
The BIOS group has developed dual-axis OCT (DA-OCT) to improve depth penetration and enable deep tissue imaging2. DA-OCT uses distinct illumination and collection apertures (Figure 3) to triangulate the signal to layers deep beneath the tissue surface. The approach provides enhanced depth penetration capabilities by allowing collection of scattered light while also increasing rejection of out-of-focus light.
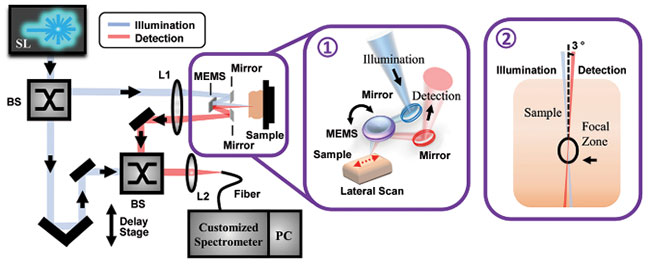
Figure 3. Dual-axis OCT (DA-OCT) provides increased depth penetration using distinct illumination and detection apertures (inset 1). The two apertures intersect beneath the tissue surface (inset 2). SL: supercontinuum laser; BS: beamsplitter. Adapted with permission from reference 2.
Such technology can provide an advantage for clinical applications in dermatology. To illustrate, Figure 4 compares DA-OCT imaging of porcine ear skin to conventional OCT imaging. DA-OCT yields lower axial resolution than conventional OCT because of the detection of multiple scattered photons. However, the epidermis and dermis can still be clearly resolved on the DA-OCT image. The depth penetration of the conventional OCT system is as expected for the depth of focus for this scheme.

Figure 4. Representative images of porcine ear skin acquired with DA-OCT and compared to conventional OCT. E: epidermis; D: dermis. Adapted with permission from reference 2.
In contrast, DA-OCT produces better depth penetration with somewhat lower resolution. However, this is not simply due to the decreased numerical aperture afforded by the wider beam. If a comparable beam waist was used in a conventional OCT scheme, the predicted depth of focus would be much larger, but scattering would preclude deep penetration. Using the DA-OCT approach, structures still can be clearly resolved and new subsurface features are seen.
Real-time speckle reduction
Since OCT is based on coherent imaging, systems often produce significant speckle (or snow) in the images. This is a fundamental aspect of OCT imaging and cannot be avoided. However, it can be reduced using hardware modifications or software post-processing. The BIOS group has developed a new OCT processing algorithm that can be applied in real time by taking advantage of the GPU that is standard on most PCs. This approach is based on a spectroscopic processing algorithm termed the dual window method3. It was originally designed to provide wavelength-dependent tissue properties that maintained the depth resolution of OCT4, but has recently been used to reduce speckle in OCT images5.
With the dual window method, OCT data is processed into distinct spectral bands. Unlike the conventional short-time Fourier transform processing approach, the dual window technique does not produce a significant loss in depth resolution3. For speckle reduction, dual window acts as a frequency compounding technique. The detected signal is subdivided into distinct spectral bands where each has a distinct speckle pattern. The various spectral bands can then be averaged to reduce speckle noise up to 25 percent (Figure 5). While this is more modest than other speckle reduction techniques6, it is a noticeable, significant reduction and can be implemented without hardware modification or the need to acquire multiple images. This speckle reduction technique can thus be used in nearly any OCT system to improve image contrast and provide clearer images.
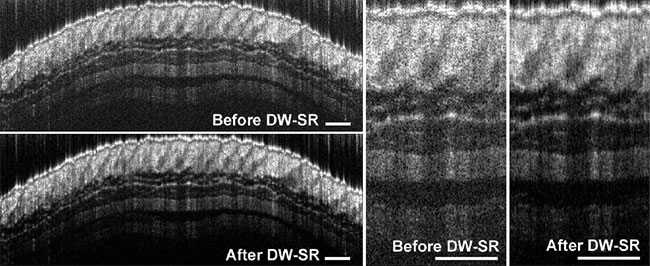
Figure 5. Representative images of healthy mouse skin before and after dual window speckle reduction (DW-SR) — five 30-nm spectral windows for DW-SR. Structural details of subcutaneous tissue can be better resolved after applying the DW-SR of 25 percent. Adapted with permission from reference 5.
It’s time for OCT to expand beyond ophthalmologist practices and hospital settings. OCT systems can be powerful, portable, and accessible, making them a better fit for doctors’ offices and low-resource settings. To broaden OCT’s use, the lower-cost solution facilitates application to other tissues, such as skin, in which the increased penetration depth of DA-OCT will increase adoption. The simple method for speckle reduction can enhance OCT images, making them easier to read and interpret.
Preparing OCT for use at the point of care focuses on advances that make the technique easier to use and more broadly applicable.
Meet the author
Adam Wax is a professor of biomedical engineering at Duke University, where he earned a doctorate in physics. He is co-founder and president of Lumedica and is a fellow of OSA, SPIE, and AIMBE; email: [email protected].
References
1. S. Kim et al. (2018). Design and implementation of a low-cost, portable OCT system. Biomed Opt Express, Vol. 9, Issue 3, pp. 1232-1243.
2. Y. Zhao et al. (2017). Dual-axis optical coherence tomography for deep tissue imaging. Opt Lett, Vol. 42, Issue 12, pp. 2302-2305.
3. F. Robles et al. (2009). Dual window method for processing spectroscopic optical coherence tomography signals with simultaneously high spectral and temporal resolution. Opt Express, Vol. 17, Issue 8, pp. 6799-6812.
4. F. Robles et al. (2011). Molecular imaging true-colour spectroscopic optical coherence tomography. Nat Photonics, Vol. 5, Issue 12, pp. 744-747.
5. Y. Zhao et al. (2018). Real-time speckle reduction in optical coherence tomography using the dual window method. Biomed Opt Express, Vol. 9, Issue 2, pp. 616-622.
6. O. Liba et al. (2017). Speckle-modulating optical coherence tomography in living mice and humans. Nat Commun, Vol. 8, Article 15845.