Optics commonly optimize the laser beam used in materials processing, but new beam-shaping methods are achieving more sophisticated and almost arbitrary beam shapes for new applications.
ANDREAS THOSS, CONTRIBUTING EDITOR
When Theodore (Ted) Maiman described the laser as a solution seeking a problem, beam shaping was not a big deal. A lens or two were enough to project the beam profile onto a screen. The main challenge was operating the laser itself.
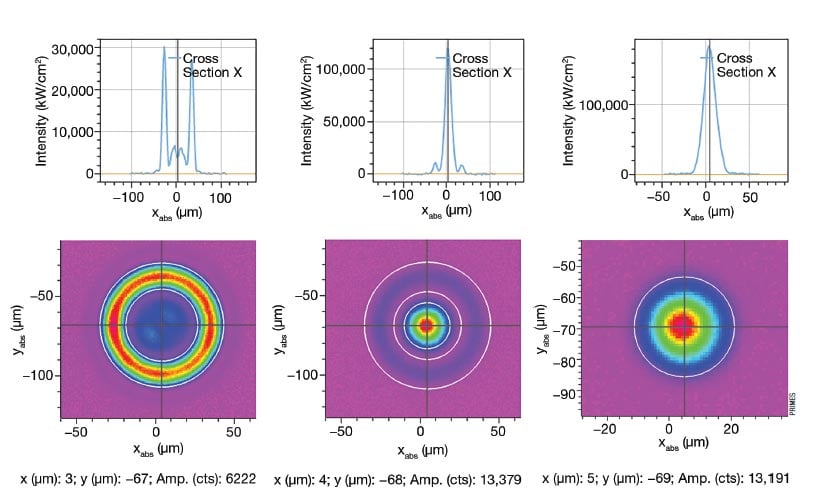
Figure 1. A tunable distribution of energy in the core and cladding of a fiber laser enables the creation of various beam shapes in real time. Three such profiles have been measured with PRIMES’ MicroSpotMonitor MSM+ HB10. Power ratios of core/cladding are 10/90 (left), 80/20 (middle), and 100/0 (right).
Courtesy of PRIMES.
This changed when laser technology
started finding problems to solve. When these lasers started to cut metal, for
example, users found that the surface roughness of the cuts depended on the
polarization direction of the beam:
Surfaces were smoother when the chosen polarization ran parallel to the direction of the cut. It became clear that adapting
the laser beam to the cutting process could make a big difference. Since then, laser optics have been developed for different laser applications. It is always the application that defines the search for a perfectly shaped laser beam. Thus, future beam-shaping technologies will need even more knowledge of the process details.
Refractive beam shaping
In materials processing applications, the first task of beam shaping is to achieve the right intensity on a workpiece to maximize processing speed and workpiece quality. The next step is to optimize the laser beam profile from common native form, such as Gaussian, into a flat-top or doughnut-shaped profile. Such transformations can be done simply with refractive optics, such as lenses or prisms. Details are very well presented on the websites of component providers, such as Edmund Optics.
Laser-processing heads with sophisticated lens systems, process gas delivery, and process-monitoring systems are also available from vendors such Scansonic, Coherent, or PT Photonic Tools.
Describing the Gaussian shape of a beam profile as natural is a bit misleading. It is indeed natural for a single-mode fiber laser, but many lasers emit beam shapes far more complicated than a Gaussian spot. Diode lasers, for example, have an elliptical profile in which the horizontal and vertical parts of the beam diverge at different rates. In the simplest case, a cylindrical microlens placed very close to the emitting facet of the diode can compensate for its elliptical profile.
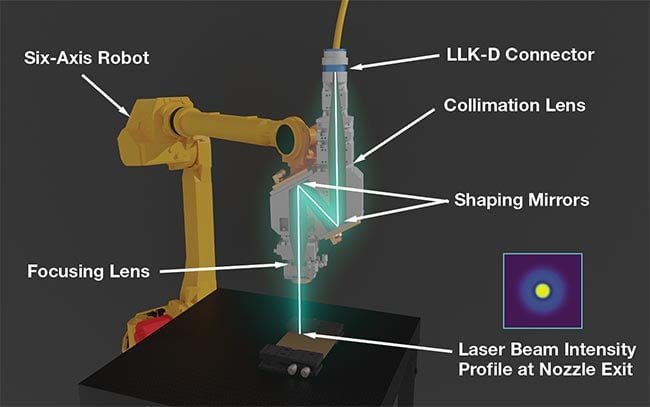
Figure 2. Cailabs engineers developed its Multi-Plane Light Conversion (MPLC) beam-shaping technology to work with ultra-intense and ultrashort pulses. The technology uses phase manipulation via a sequence of reflections as the beam travels back and forth between two mirrors, one of which has a textured surface. The beam profile (inset) has 70% of its energy in the central spot and 30% in the ring. The technology was tested with TRUMPF’s 8-kW TruDisk 8001 for copper welding.
Courtesy of Cailabs.
Excimer lasers start with a rectangular beam profile, where the long axis has a top hat shape and the short axis has a Gaussian-like shape. These UV lasers require more effort to make their beams uniformly round. Special micro-optics can often achieve this only within a
certain plane of the beam. Further,
excimer lasers often need beam homogenization, in which the beam is sent through a diffuser and focused afterward.
For diode and excimer lasers, the beam-shaping optics are usually part of the beam source, providing users with a desired beam profile “out of the box.”
Laser systems may also incorporate additional static systems that adapt the beam profile into a shape more suited to the particular application. Excimer lasers, such Coherent’s LineBeam series, for example, enclose optics that shape their rectangular profile into a line that measures 0.6 × 1500 sq mm for display manufacturing. This is a special form of a top hat profile.
Variable fiber optics
Obviously, fixed optics shape a beam for
a largely fixed use. This can be quite limiting if the task changes. If a laser is used for process development, then the optimal beam shape may not even be known.
These challenges demand variable
optics, and some specialized solutions have entered into the market in recent years. Not surprisingly, they are adapted to fiber lasers, the most popular sources for materials processing applications. These special optics allow users to tune the beam into different shapes, from a pure Gaussian spot to an approximate flat top, to a doughnut-shaped beam. The various providers of the technology take different approaches to achieve this effect, which leads to some differences in the performance of the beam-shaping optics.
The company nLight, for example, developed its Corona technology to
enable flexible all-fiber beam shaping. The technology offers single- and multi-mode laser options, each with different tuning ranges. For example, it allows users to tune a 100-µm spot into 200- or 300-µm rings. The technology relies on distributing the laser energy between the core and the cladding of the fiber1.
Coherent’s Adjustable Ring Mode (ARM) technology essentially allows users to independently tune the power in the core and the cladding of a fiber laser. This enables generation of a high-brightness center beam surrounded by a multimode beam that can be modulated separately.
This capability has a positive influence on copper welding applications. But
collectively, these new active beam-shaping technologies for fiber lasers have many uses. For laser cutting applications, they allow quick transitions between the initial piercing of the sheet and the actual cutting process. Or they can mitigate the compromise between speed and edge quality in cutting applications.
For welding, tunable beam-shaping technology can help reduce spatter. In 3D printing of metals, it offers rapid changes between coarse and fine structure buildup. This technology is available from many suppliers and is not limited to fiber lasers as TRUMPF has demonstrated with the BrightLine optics for its disk lasers.
New ideas take shape
So far, fiber-based beam-shaping
techniques are offered only for radial symmetric light distributions. They cannot do much on the beam’s z-axis. Some applications are more demanding and require features such as a Bessel beam
(a beam with an elongated focal region)
or a multi-beam profile.
Such problems can be solved with diffractive optics that retard the phase of the beam’s wavefront. In the far field, the wavefront, and therefore the intensity distribution, is transformed into a new shape. This is achieved using diffractive optical elements (DOEs), which are glass plates with diffractive structures etched into them. These patterns are fixed and imprint a new phase structure onto the wavefront. With a DOE, one can create Bessel beams, such as those used for cutting curved contours, into glass. DOEs are also used as beamsplitters or to create multi-beam patterns. Researchers from the Fraunhofer Institute for Laser Technology (ILT) have used this idea to create an 8 × 8 beam pattern out of a 1-kW ultrashort-pulse laser. They applied the pattern to drill holes during the fabrication of wastewater filters.
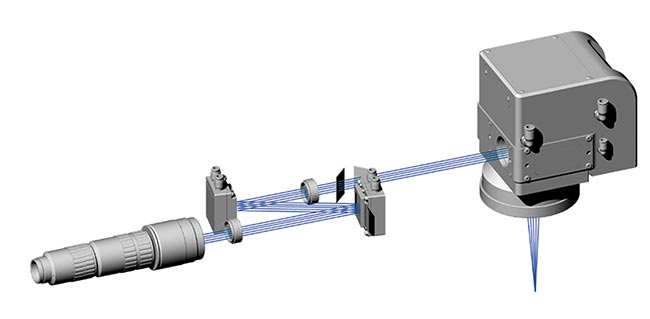
Figure 3. A dynamic beam-shaping setup based on two cascaded spatial light modulators is currently being investigated under the EU-funded Metamorpha project, which aims to develop an agile micromachining platform based on ultrashort-pulse laser beams tailored in amplitude and phase. Courtesy of Fraunhofer ILT.
French laser maker Cailabs has also
developed a beam-shaping technology
that can be used for ultra-intense and ultrashort pulses. The company’s Multi-Plane Light Conversion (MPLC) technology uses phase manipulation via a sequence of reflections. The beam travels back and forth between two mirrors, one of which has a textured surface. The MPLC technique can take one or more beams at input and output and can have either fibered or free-space inputs and outputs.
The reflective solution imposes no losses and also enables mode cleaning as MPLC technology can distinguish different modes on the beam’s path between the mirrors.
For materials processing solutions, Cailabs claims that its technology produces flat-top profiles that have more stability and a larger depth of focus compared with profiles from conventional DOEs. This allows higher throughput for various kinds of surface-processing procedures.
MPLC and DOE solutions both rely on static structures. For more flexibility, one can also use spatial light modulators (SLMs) for tunable phase manipulation of wavefronts. This can be done with SLMs based either on liquid crystal on silicon (LCoS) or MEMS. Either offers a tunable array of phase retarders. Additional degrees of freedom can be provided by cascading the modulators.
Carlo Holly, a professor of Optical Technologies at RWTH Aachen University in Aachen, Germany, is developing such systems in close cooperation with Fraunhofer ILT. “Systems with cascaded phase masks enable robust beam shaping,” he said. “That means the beam can be misaligned with respect to the optical system without changing the beam shape.”
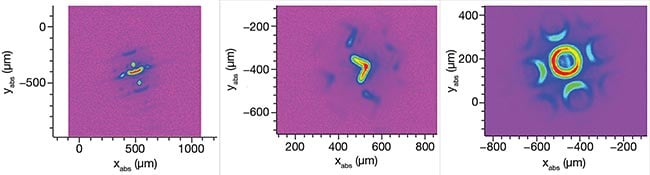
Figure 4. Civan Lasers’ coherent combination of similar but phase-modulated beams can create arbitrary beam profiles as shown in these three measurements captured by BBW Lasertechnik. Courtesy of BBW Lasertechnik.
Holly has developed software routines based on artificial intelligence that calculate the settings of the modulator cascade to create a certain beam shape. This enables precise and highly flexible beam shapes with variation of the profile along the beam’s path of propagation. It can also increase the effective depth of field for special beam profiles.
The dynamic character of LCoS allows changes to beam shape, even within a running process. That allows for application-adapted beam profiles as well as a feedback loop during processing. This capability is currently being further investigated in the EU-funded Metamorpha project, which aims to develop an agile micromachining platform based on ultrashort-pulse laser beams tailored in amplitude and phase. The beams measure the surface of a workpiece and use an artificial intelligence routine to create a beam profile that is perfectly adapted to the surface and the process. The goal is to ensure that the end result is “first time right,” Holly said.
Building a beam from scratch
An entirely new way of adapting laser beams to an application is through the coherent combination of similar but phase-modulated beams. This is the approach taken by Israel-based Civan Laser. The company’s systems take a single-mode beam, split it into a number of identical replicas, amplify and modulate those replicas, and finally recombine them together coherently — meaning the recombined beams are phase-matched. Their electromagnetic waves are related to each other and thus the electromagnetic fields of the single beams can be added or subtracted. Noncoherent beams can only add amplitudes or intensities, but neither subtract nor add phases.
The phases are modulated and, although the combining optics are fixed, the sum of the phase-modulated beams forms a new beam profile in time and space.
With its so-called Dynamic Beam Laser technology, Civan’s sources can create almost arbitrary beam profiles, with possible changes in the megahertz regime. Beam sequencing as well as beam steering also becomes possible.
BBW Lasertechnik in Prutting, Germany measured various beam profiles enabled through Civan’s technology laser using a MicroSpotMonitor MSM+ HB10 from PRIMES. The beam shapes are as arbitrary as promised. Users have noticed, however, that there can be residual light around the actual beam profile that contains significant amounts of power. If you have a 10-kW beam of which 5 kW is in your main profile, then the remaining power is scattered around.
Summary
Some sixty years ago, the laser was a solution in search of a problem. Times have changed. Lasers are sophisticated tools that solve many tasks in manufacturing today, and the question of which laser to apply to a task largely depends on the kind of job it is supposed to run.
Shall it be just one repetitive process in a huge factory? Or is the laser supposed to solve various tasks in a small job shop? The former can rely on lasers optimized for a single process. The latter will require something more versatile.
In large manufacturing settings, the process will define the requirements for beam shaping. Thus, process knowledge is key to finding the right solution. A team from TRUMPF and Fraunhofer ILT visited the Positron-Electron Tandem Ring Accelerator (PETRA) facility in Hamburg, Germany, for this very purpose. PETRA is part of Germany’s DESY electron synchrotron facility where it generates very fine pulses of strong x-rays. The TRUMPF and ILT team used these rays to image the welding process of copper electrodes at more than 10,000 frames per second. TRUMPF experts took the resulting process knowledge to improve copper welding processes with its green lasers.
Not everybody can take their research this extra mile. But it illustrates how the selection of a laser system can be optimized today: When the process is well understood, then a process window can be defined, and the laser can be optimized to match this window with appropriate optics. If the process is not known or needs a varying profile, then tunable beam shapers can provide a solution or a beam can even be developed from several separately shaped beams. Almost any beam shape can be created today. In the end, costs and quality may be the main criteria to define which beam-shaping solution is best for a given process.
Reference
1. B. Wedel. (2023). The next generation of
tactile laser processing heads. PhotonicsViews, Vol. 20, No. 5, pp. 33-35.