Nanosurgery is forever hitting the headlines
as research groups around the world explore the myriad applications for this precise
technique. Couple this to the development of lasers with ever shorter pulse durations,
and it’s little wonder that nanosurgery is creating quite a buzz in laboratories
the world over.
Today, nanosurgery is employed mostly as an in vitro technique
for cell and tissue manipulation and as an in vivo technique in model organisms.
However, with future developments of hybrid technologies, such as pairing laser
sources with imaging systems and developing safe and reliable biological methods
for in vivo operation, nanosurgery is set to become a formidable method in medicine.
Possible applications include gene therapy, nerve regeneration and cancer treatment
involving the selective damage of tumoral cells.
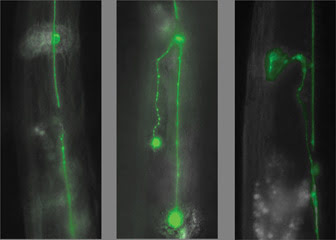
High-throughput in vivo femtosecond laser surgery allowed discovery of drug leads that enhance neuronal regeneration. The first two panels show dramatic enhancement of axon regeneration
when subjected to two potent chemicals with respect to a control (far right) without
any chemical. Courtesy of Yanik Lab at MIT.
The first observations at the nanoscale were achieved nearly 80
years ago with the advent of electron microscopy in 1931. Manipulation at such a
scale, however, had to wait another 25 years for the surgical removal of subcellular
organelles (mitochondria) in the 1950s. The early 1960s saw the invention of the
first lasers and, since then, optical manipulation at the nanoscale has become a
key technology in many new fields of research.
Some of the main application areas include nanomechanics in surface
physics, nanoelectronics in quantum computation, nanofabrication in materials science,
molecular dynamics in biology, cellular and subcellular manipulation, and tissue
engineering, among others.
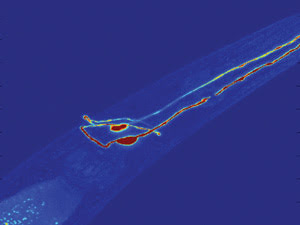
A femtosecond laser severed the dendrite in this AFD neuron, which is the primary
type of neuron underlying thermotactic behavior. Courtesy of Samuel Chung/ Mazur
Group, Harvard University.
Precise nanosurgery ensures that nothing other than the targeted
structure is damaged within the cell or organism. This allows scientists to probe
and study important subsequent biological processes. For example, in groundbreaking
research currently under way at MIT in Cambridge by associate professor Mehmet Fatih
Yanik and at the University of Texas at Austin by associate professor Adela Ben-Yakar,
nanosurgery is being used to sever neural processes and to observe nerve regeneration.
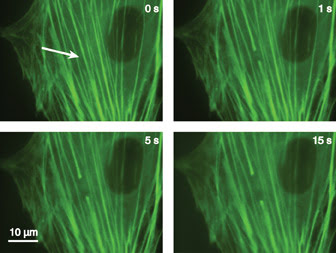
Shown is an actin filament retracting over time after ablation with
a femtosecond laser. Courtesy of Iva Maxwell/Mazur Group, Harvard University.
“Nanosurgery allows the ablation of structures of sizes
as small as 300 nanometers like actin filaments, cytoskeleton, cisternae of endoplasmic
reticuli for cellular organelles or axons, dendrites or synapses for neural processes,”
said Ben-Yakar, who is also the director of FemtoLab (FEMTOsecond Laser-Assisted
Biomedicine). “It goes without saying that collateral damage is not an option
when surrounding tissue may actually provide a structural cue to regeneration.”
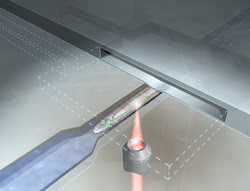
Shown is a model of femtosecond laser nanosurgery-
on-a-chip for nerve regeneration studies in vivo using a whole organism, C. elegans.
The chip allows chemical-free immobilization of the worm for precise laser nanosurgery
of its axons. The full automation of this chip now provides the possibility for
high-throughput screening of genes affecting nerve regeneration. Courtesy of Ben-Yakar
Group.
Nanosurgery advances
Progress in nanosurgery currently pursues two avenues of research:
One path relies on mechanical and robotic tools with nanoscale cutting precision,
while the other relies on optical methods, which include taking advantage of the
unique properties of lasers and their interaction with plasmonic nanoparticles.
“Researchers are investigating several scientific avenues
to achieve mechanical and robotic tools for nanosurgery, from plasma jets to nanorobots,”
said Ben-Yakar. “Each method offers specific applicability, depending on which
organs are being operated on.”
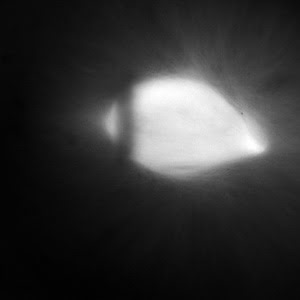
A femtosecond laser cut these microtubules, causing their depolymerization
in mitotic spindle (left). Courtesy of Valeria Nuzzo/Mazur Group, Harvard University.
Advances in ultrashort pulsed laser technology provide an attractive
alternative to chemical and mechanical approaches for cell and tissue manipulation.
Indeed, femtosecond laser ablation, in addition to being a noninvasive and reliable
technique, can be used to perform very accurate and selective surgery, as the nonlinear
laser-material interaction is confined to the focal volume. For instance, a cell
organelle can be dissected while leaving the cell membrane intact. What’s
more, femtosecond irradiation ensures nanoscale precision as well as reduced mechanical
and thermal effects.
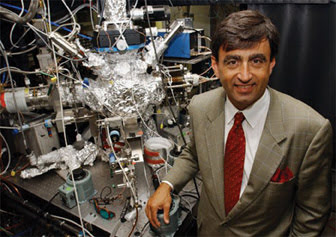
Eric Mazur, Harvard University
physics professor, discusses developments in ultrafast lasers. Courtesy of Stephanie Mitchell/Harvard University News Office ©2002.
“The last two decades have witnessed strong advances in
laser technology for decreasing pulse duration and increasing pulse energy,”
said Eric Mazur, professor of applied physics at Harvard University in Cambridge,
Mass. “Among them are the development of the titanium:sapphire crystal and
rare-earth-doped glass as lasing materials for the generation of very short pulses,
and the introduction of the chirped pulse amplification technique for safely increasing
the pulse energy.
“These developments have made ultrafast lasers more accessible
in terms of cost and maintenance, enabling short-pulsed lasers to be used in research
as well as in clinical settings. In parallel with laser advancements, on the biological
side, the cloning of the green fluorescent protein has been extremely useful in
localizing particles targeted by the laser,” he added.
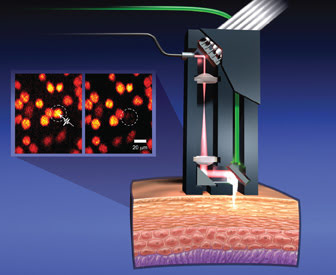
A clinical endoscope is used to perform image-guided femtosecond
laser microsurgery of cancer cells. The inset shows two-photon fluorescence images
of cancer cells taken with the prototype (left image) and the same region after
the targeted ablation of a single cell. The targeted cell was instantly destroyed,
while neighboring cells were left intact. Courtesy of Ben-Yakar Group, University
of Texas, Austin.
Nerve regeneration
According to Yanik, who heads up the High-Throughput Neurotechnology
Group at MIT, some of the biggest breakthroughs have been in the innovative applications
of nanosurgery for various investigations that would otherwise be impossible. “Since
most cellular processes vary on nanometer-length scales, optical manipulation at
nanoscale resolution opens up a new dimension in cellular and subcellular scale
investigations,” he said.
Working together in 2004, for instance, Yanik and Ben-Yakar used
nanosurgery to demonstrate the first study of neuronal regeneration in a small multicellular
organism (Caenorhabditis elegans). Several biology labs have subsequently adapted
the technique, and in the last C. elegans meeting, there were several novel findings
made possible by nanosurgery.
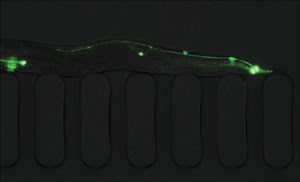
High-throughput microfluidics captured and immobilized the C. elegans seen in this screening chip. Neurons that are labeled by a green fluorescent protein reporter are visible inside
the optically transparent worm. Courtesy of Yanik Lab at MIT.
In 2007, Yanik’s group developed a high-throughput microfluidic
chip technology to manipulate C. elegans. Subsequently, in 2008, teams under Yanik
and Ben-Yakar independently demonstrated a microchip that can rapidly perform nanosurgery
on C. elegans. Yanik’s technology also allowed high-throughput in vivo neuronal
regeneration screens using large drug libraries and genetic factors affecting neuronal
regeneration. Since then, the Yanik group has discovered highly potent drug leads
that enhance neuronal regeneration. The lab is now testing these on human neurons
derived from stem cells.
More recently, the Ben-Yakar Lab developed a fully automated microfluidics
chip that can perform femtosecond laser nanosurgery of axons without human intervention.
This new automated platform now allows genome-wide screening of nerve regeneration
in a high-throughput manner.
“This microfluidic device allows us to precisely manipulate
C. elegans inside small channels and eliminates the need for anesthetics, which
have shown to interfere with the axonal regrowth process,” Ben-Yakar said.
“With tools such as laser nanosurgery and microfluidic devices and their full
automated integration, we can undertake gene and drug screenings that usually need
high-throughput systems because of the sheer amount of samples involved. Whole-genome
screening is now conceivably possible within two years.”
Cancer treatment
The ability to selectively and precisely ablate biological tissue
is a natural candidate for the treatment of cancer. In this field, nanoparticle-assisted
surgery activated by lasers shows great potential.
Nanoparticles conjugated with specific molecules (for example,
ligands or antibodies) can anchor onto specific cells or tissue. Once exposed to
a laser beam, the nanoparticles act as lenses and heat and/or ablate the targets
they are attached to, such as cellular membranes or mitochondria.
“Plasmonic laser nanosurgery is a novel photodisruption
technique that exploits the large enhancement of femtosecond laser pulses in the
near-field of metal nanoparticles for the selective and nonthermal nanoscale manipulation
of biological structures,” Ben-Yakar said. “We have demonstrated the
feasibility of plasmonic laser nanosurgery for both cellular death by necrosis for
cancer treatment and transient pore formation for cellular transfection.”
Precise imaging needed
According to Yanik, a promising line of research that is yet to
be explored is the use of coherent ultrafast pulse-shaping techniques to increase
precision, chemical selectivity and repeatability of nanosurgery. But perhaps the
most important work needs to be done in the field of microscopy.
“Nanosurgery is a blind operation without superresolution
microscopy,” Yanik said. “Thus, I believe there is significant need
to combine nanosurgery with new microscopy techniques such as STED (stimulated emission
depletion microscopy), stochastic optical reconstruction microscopy/photoactivated
localization microscopy and structured imaging.”
In fact, the MIT group recently proposed the use of electron beams
for noninvasive molecular-scale imaging, which it hopes may one day be used to simultaneously
image and perform molecular-scale surgery with electron beams.
Nanosurgery research may still be in its infancy, but with so
many exciting breakthroughs in the field, there can be little doubt that this mighty
tool is coming to a surgery table near you.