What’s Next for Biological Applications?
Around the world, researchers are developing nanoscale devices that receive and emit light. These devices could work better than existing methods of detecting biological molecules for research and direct medical diagnostic applications.
Researchers at the University of Illinois at Urbana-Champaign have developed a nano-size needle that can penetrate the cell membrane. The nanoneedle enabled them to deliver fluorescent quantum dots directly into cells for live-cell imaging. The device also could be used for purposes such as drug delivery or for probing the electrochemistry of a cell.
The nanoneedle is approximately 50 nm in diameter and consists of boron nitride fused to the end of a glass pipette and coated with a thin layer of gold. Linker molecules connect the molecular cargo to the nanoneedle.
When the nanoneedle goes inside the cell, the molecular cargo is released, allowing the researchers to precisely control and monitor the release of the fluorophore. The precision will allow them to release and track the action of single molecules in cells, something that is certainly desirable but also difficult to do. This work is detailed in the May 13, 2009, issue of Nano Letters.
Staying focused
The most familiar antennas operate at radio frequencies. When an antenna is fashioned on the nanoscale, it can function in the optical regime.
A multinational group of researchers has used appropriately called “gap antennas” – metal nanorods with gaps in the center that concentrate infrared light. Their ability to focus infrared light can be used for sensing applications in biological experiments. The nanoantennas also could detect light very rapidly for applications such as medical diagnostics and information processing.
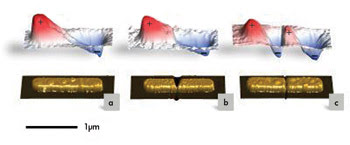
When fabricated with gaps of varying structures, the metal nanorods pictured in the bottom row act as “gap antennas” that focus infrared light in different ways, as shown in the graphs above. Courtesy of Martin Schnell of CIC nanoGUNE.
The researchers added metal bridgework inside the gap just to see what would happen. They found that the bridgework could change the field around the antenna dramatically.
Some of the researchers previously had developed a scattering near-field microscope with dielectric tips. This device enabled them to probe the field surrounding the nanoantennas.
The researchers also created simulations of the fields that agreed with the experimental data. They pointed out that these simulations are useful because predictions can be made about the operation of the antenna, depending upon the material in the gap or the lack thereof.
The work with this gap antenna is significant because there may be some cases in which altering the gap is more desirable than changing the length or shape of the antenna itself. This study is described in the May 2009 issue of Nature Photonics.
The researchers came from various institutions in San Sebastian, Spain, including CIC nanoGUNE, Donostia International Physics Center DIPC and Centro de Fìsica de Materiales of CSIC/UPV-EHU. Other institutions involved with the work included Harvard University and the Max Planck Institute of Biochemistry in Munich.
Tune in, turn on
Some researchers are developing gold nanospheres for sensing applications. That is the case for the Jin Zhang lab at the University of California, Santa Cruz.
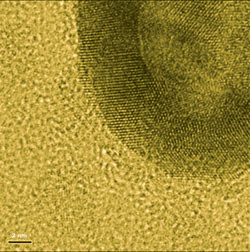
Pictured is a partial view of a hollow gold nanosphere as seen under an electron microscope. These nanospheres, activated by surface-enhanced Raman spectroscopy, were demonstrated in mice to be useful for photothermal treatment of skin cancer. Courtesy of Adam Schwartzberg and Jin Zhang. The image has been colorized.
The Zhang lab can adjust the optical properties of the hollow, tiny gold balls by varying the size and thickness. With reliable repeatability, the researchers can synthesize the particles with diameters from 20 to 70 nm, the ideal size range for entering cells.
The gold spheres absorb visible and near-infrared light with intense, narrow absorption peaks. In fact, the hollow nanospheres are 50 times better at absorbing near-infrared light than their solid counterparts. They originally were developed for surface-enhanced Raman scattering, an optical technique that can detect biological molecules.
In their most recent work, the researchers, in collaboration with the Chun Li lab at MD Anderson Cancer Center in Houston, used the infrared absorption properties of the nanospheres to kill tumor cells.
The researchers attached tumor-targeting peptides to the gold nanospheres and injected the nanospheres in mice with skin cancer. They focused a near-infrared laser beam on the skin of the mice, which heated the gold nanospheres and the tumor cells to which the nanospheres were attached. The researchers reported this study at the American Chemical Society annual meeting March 22, 2009, in Salt Lake City.
The Zhang lab is experimenting with other nanostructures. His graduate student, Tammy Olson, has created similar structures of silver and gold that have even greater surface-enhanced Raman spectroscopy activity.