Simple, fast and particularly cheap – is it any wonder that molecular spectroscopy is quickly becoming one of the most common tools in the laboratory?
Molecular spectroscopy is photonics at its most fundamental: the interaction between light and matter. The information that this technique uncovers is also fundamental to us and to how we relate to every other organism around us. From routine analysis to specialist testing, molecular spectroscopy is used in chemistry, pharmacy, medicine, food control, environment, the life sciences and many other areas.
Measuring the energy or wavelength spectrum of light that is incident, scattered and/or emitted from molecules can reveal a whole host of inner biological workings. UV and visible radiation often are used on liquid samples, but the analysis of solid and gaseous samples is also possible using special accessories.
“Thanks to the wide range of different spectrophotometer models and accessories, molecular spectroscopy can be used in almost every field of application in research and development or routine analysis,” said Alexandra Kästner, product specialist for molecular spectroscopy at Analytik Jena AG in Jena, Germany.
The industrial manufacture of the world’s first analytical instruments began in Jena in 1846, in the Carl Zeiss GmbH optical workshop. Carl Zeiss launched the world’s first spectrometer in 1874, based on Ernst Abbe’s scientific theories for producing optical measuring instruments.
Analytik Jena was founded in 1990, and five years later it acquired lab analysis technology from Carl Zeiss Jena GmbH. This was a turning point that allowed Analytik Jena to act as a developer, manufacturer and provider of innovative high-tech products.
Accessories extend applications
Some of the most common applications of molecular spectroscopy include the quantitative analysis of the concentration or the kinetics of various substances. Qualitative measurements for identifying and determining the purity of various substances are also commonly made using molecular spectroscopy.
But the list of applications is expanded by combining molecular spectroscopy with other technologies.
With special reflectance accessories and software tools, it is possible to measure the color of different kinds of samples or to analyze film thicknesses and reflectance properties of coatings of solid materials.
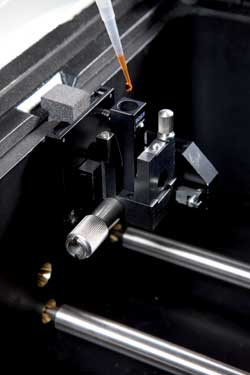
The Specord Plus is an adjustable cell holder with an ultramicrocell for very low sample volumes.
“Besides the high technical performance of spectrophotometers, the wide range of accessories for different kinds of applications and samples was adapted to different requirements regarding sample throughput, cost and time reduction, and to optimize the lab routine,” Kästner said. For example, particularly in the life sciences, scattering samples with high optical density or very low sample volume are a special challenge.
“Thanks to special adjustable cell holders in combination with special cells, measurements of samples with a volume of only 5 µL can be carried out very easily, quickly [and] with a high accuracy,” she said. “But, also, time-controlled measurements such as enzyme kinetics that require an accurate temperature can be performed absolutely precisely by means of Peltier-controlled cell changers.”
Temperature changes and other environmental variables are a constant challenge to those working with biological samples where maintaining sample integrity is critical. Accurate temperature control often is provided by a Peltier cell changer, whic often will include some sort of temperature controller.
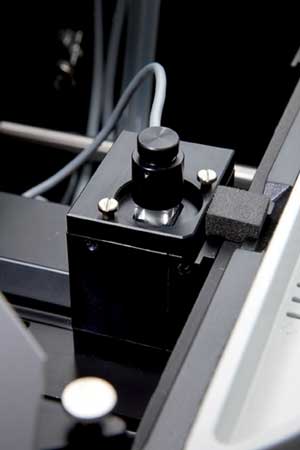
Accurate temperature control often is provided using a Peltier cell
changer, such as this Peltier-controlled cell holder from Analytik Jena
AG. Images courtesy of ©Analytik Jena AG.
A Peltier cooler is a solid-state active heat pump that transfers heat from one side of the device to the other, allowing cooling to below ambient temperature with an accuracy of around a tenth of a degree.
Fluorescence spectroscopy
When it comes to many biologically relevant molecules, which often have nanoscale features, reflected or transmitted light does not have the resolving power to distinguish its finer points. This is due to the diffraction limit as well as to the lack of natural optical contrast that could be used to help parse this information.
Fluorescence allows us to probe the environment around special molecules to ask what is going on, said fluorescence applications scientist Dr. Jeremy Pronchik of Horiba Scientific. The Irvine, Calif.-based company is a scientific instrument maker for a vast array of industries, including automotive, process and environmental monitoring, in vitro medical diagnostics, semiconductor manufacturing and metrology. “A battery of fluorophores are available that are sensitive to polarity, pH, temperature, hydrogen bonding, viscosity, polarizability, the presence of specific molecules and ions, proximity to other fluorophores and confinement,” Pronchik said.
“These properties characterize both the variability within and amongst biological macromolecules, the environments in and around cell compartments and membranes, and the solution conditions applied by scientists to perturb their system.”
Each fluorophore has a particular brightness, lifetime and resistance to photobleaching, and responds to a particular range of wavelengths. This extensive list of features allows researchers to tailor a reporter to the needs of the experiment as well as to the limitations of their equipment. “A well-characterized fluorophore allows access to a wealth of information about the molecular systems that govern our lives,” Pronchik said.
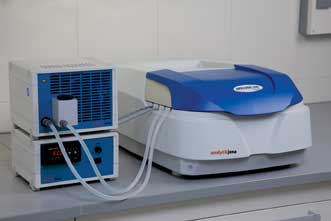
The Specord Plus is a Peltier-controlled cell holder with a temperature control unit and an external heat exchanger.
One of the most recent emerging trends, he noted – particularly in fluorescence spectroscopy – is to measure the lifetime of emitted light.
Unlike Raman, which has rich information in the peak positions, most fluorescence spectra are broad, and researchers typically rely on the contrast in intensity or lifetime of several measurements in which an experimental parameter (e.g., temperature, pH, ionic strength, concentration of quenching agent, chaotrope, binding partner or crowding agent) is modulated. Steady-state fluorescence intensity can be confounded by photobleaching, and the lifetime can be more robustly observable.
Future growth
Much of molecular spectroscopy will look the same in the next few years, Pronchik predicts. However, the way in which information is extracted from the data is set to improve.
“Modern instruments are already capable of collecting high-resolution data, and in the last few years, we have seen things like PARAFAC [parallel factor analysis] and the array of superresolution microscopy techniques reduce and enhance large data sets,” he said. “The goal is to provide contrast in an experiment so that small changes become readily apparent.
“The field is becoming very clever in taking the same data we have been looking at for years, turning it inside out and sideways, and revealing changes that our eyes were never able to see.”
A noticeable development has been in shrinking the large TCSPC (time-correlated single-photon counting) optical tables to less expensive small benchtop instruments. This is largely due to diode light sources that are stable and require no tuning or adjustment as well as to timing boards that are connected to computers with USB cables.
Scientific understanding of metal-enhanced fluorescence also is developing. “This technique produces longer lifetimes, increased intensities, decreased photobleaching rates, and increased directionality of fluorescence,” Pronchik said. “It has not found its way into mainstream molecular spectroscopy yet, but is one to keep an eye on.”
With all of the technological advances, molecular spectroscopy has been opened up to a lot of novice users, particularly in molecular biology.
While improvements continue in light sources, detectors and electronics with regard to cost, size and – most importantly – ease of use, Pronchik cautions that we may be reaching a communication plateau.
“It is a very exciting time for the field, as a lot of new ideas are being generated from this influx; however, the challenge
is to integrate them with the strong technical knowledge of the veterans,” he said. “Efficient collaborations and clear language that can cross disciplines can help new ideas mature, since there will be few researchers who have both a deep knowledge of molecular biology and photophysics.”
Top biorelated applications of molecular spectroscopy
• Concentration and purity determination of DNA/RNA and proteins.
• DNA melting point determination.
• Kinetic measurements for the determination of enzyme concentration: The change in concentration of a reactant with photometric properties determined over a certain period allows the rate of a reaction to be evaluated.
• Microscopy with extrinsic fluorophores: Dye molecules indicate the location and trafficking of important substances in the cell and across the membrane. (The discovery of green fluorescent protein and the development of the rest of the fluorescent protein family have pushed this field forward.)
• Molecular binding is determined by ELISA (enzyme-linked immunosorbent assay), in which an absorbing or fluorescing species reports a positive result. (But surface plasmon resonance provides greater information without labeling and may slowly replace the technique.)
• Other common experiments exist that require more analysis but yield valuable information: Rotational rate of a molecule and, therefore, its size or local viscosity can be determined through fluorescence anisotropy.
• Nanometer-distance information can be calculated using FRET (Förster resonance energy transfer) and multiple fluorophores.
• Counting and diffusion rate information can be extracted from FCS (fluorescence correlation spectroscopy).
• TRES (time-resolved emission spectra) can separate multiple spectra based on lifetime, or can reveal fast dynamic information or the mechanisms of excited-state reactions such as proton transfer.
• Excitation-emission matrices with PARAFAC (parallel factor) analysis can separate multiple fluorophores.
• Quantum yield experiments determine the quantum efficiency of a fluorophore.