Low-level light therapy has demonstrated clinical efficacy in a number of therapeutic areas. Yet despite over 60 years of supporting research, the scientific community is still reluctant to implement its clinical use.
RYAN SPITLER, STANFORD UNIVERSITY SCHOOL OF MEDICINE
The field of low-level light therapy (LLLT) emerged soon after the invention of the ruby and helium-neon (HeNe) lasers in the early 1960s. Since then, the technique has progressed, proving effective for a number of therapeutic areas, including reducing inflammation, promoting wound healing, reducing pain and for improving skin conditions and local circulation. However, despite decades of supporting research and a large body of evidence, the scientific community is still reluctant to implement LLLTs for clinical use.
There is a large and growing body of evidence in the scientific literature describing the beneficial effects of LLLT (Table 1). This type of therapy can be applied to a wide range of potential clinical settings, including dentistry, dermatology, rheumatology and physiotherapy. There has also been some work using LLLT for the treatment of serious neurological conditions, including traumatic brain injury, stroke, spinal cord injury and degener ative central nervous system diseases. LLLT has also been used in elective cosmetic applications, such as hair regrowth and body contouring.
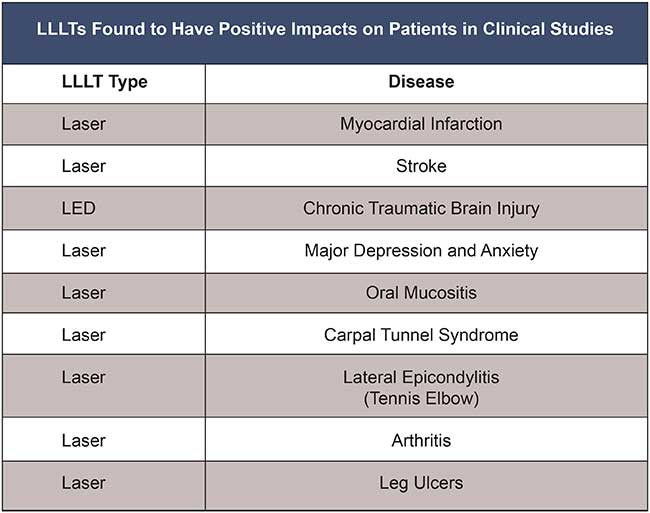
Table 1. There is a large and growing body of evidence in the scientific literature describing the
beneficial effects of low-level light therapy (LLLT). Based on information from H.Chung et al. (2012).
The nuts and bolts of low-level laser (light) therapy. Ann Biomed Eng, Vol. 40, Issue 2, Table 4. Courtesy of BioPhotas Inc.
Clinical LLLT barriers
Some of the primary barriers for LLLT’s clinical use include the various settings in which it may be applied, difficulty reproducing these settings by other investigators, uncertainty of the biochemical mechanisms triggered by the light involved, as well as the selection of an effective light delivery device. The key to achieving positive clinical outcomes is an understanding of the clinical settings involved and how to implement them for the desired application.
There are a number of light energy products on the market that provide various ranges of power output, wavelength and pulse frequencies, including the Celluma by BioPhotas Inc. in Tustin, Calif. (Figure 1); the Omnilux revive by Photo Therapeutics Ltd. in London; and the Dermaclear blue and red LED acne light treatment lamp by Britebox in Steubenville, Ohio. These products also deliver light energy in both short and long time periods. To achieve effective LLLT, the light energy being emitted must be absorbed by the target tissue.
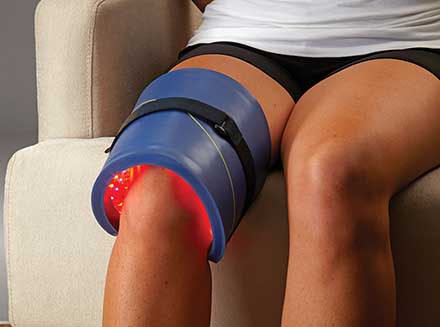
Figure 1. The Celluma light therapy technology by BioPhotas Inc. can be used to treat arthritis, muscle spasm, muscle/joint pain and stiffness, muscle tissue tension, diminished local circulation, and for post-surgical healing. Courtesy of BioPhotas Inc.
Light absorbance in tissue
Whenever light is delivered to a biological tissue, a portion of the light will become absorbed, reflected, scattered or transmitted. While the tissue will absorb the majority of the light emissions, some of the light will be reflected, due to the change in the refractive index between the air and tissue interface. Some light will also scatter. Depending on the biological tissue, the distribution of light intensity in the tissue will change. The remaining light that is neither absorbed, reflected nor scattered is then further transmitted. The light delivery process must be carefully modulated from the source device to the treatment area to achieve the desired dosing of light.
It is clear that the beneficial effects resulting from LLLT exhibit a biphasic dose response. This means that there is indeed a threshold at the upper and lower limits of LLLT leading to a positive biological response, no response or a negative response. This being said, there is no known finite set of parameters for effective LLLT dosimetry, but rather a range of effective parameters. Nevertheless, a sufficient amount of light energy must reach the target tissue in order for positive clinical outcomes to occur. Thus, in order to achieve clinical efficacy, an understanding of the mechanisms involved should be considered.
Photo-accepting molecules
LLLT is known to have a wide range of effects occurring at the molecular, cellular and tissue levels with modes of action differing depending on application. However, for the light energy to be harnessed, there must be some photo-accepting molecule with sufficient overlap within its absorbance spectrum to the light being emitted by the delivery device. The multimolecular complexes of the electron transport system may be the light receptors, and increased mitochondrial activity of complexes I, II, III and IV have been observed. Cytochrome c oxidase, part of complex IV, is generally accepted as the primary photo-accepting molecule. The absorption of this light energy then results in changes in reactive oxygen species (ROS) as well as increased adenosine triphosphate (ATP) production. Increases of intracellular ROS and ATP activate transcription factors, which lead to the activation of downstream signaling cascades, resulting in the beneficial effects observed.
There are several transcription factors that are regulated by changes in the redox state, which is the transfer of electrons between chemical species resulting in either reduction or oxidation and, in this case, leading to the formation of ROS. The transcription factors then lead to protein synthesis, which stimulates such downstream effects as increased cell proliferation, migration, modulation of the levels of cytokines, growth factors, inflammatory mediators and increased tissue oxygenation. These signaling cascades then activate stimulatory and protective genes associated with anti-aging, enhanced wound healing, reduced pain and improved skin conditions as well as other clinical benefits. While these areas of research are still actively under investigation, they are relatively well-characterized.
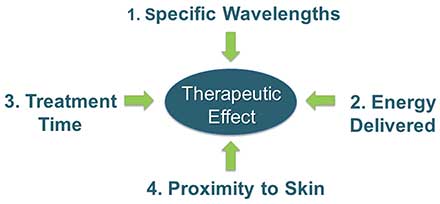
Figure 2. The potentially complicated optical parameters can be simplified into four distinct components: 1. Specific wavelengths being used; 2. The amount of light energy being delivered; 3. The duration of each individual treatment as well as the treatment regimen; and 4. The proximity of the light source to the treatment area. All of these factors must be carefully considered and accounted for appropriately in order for successful treatment outcomes to occur. Courtesy of BioPhotas Inc.
Wavelength, energy density and treatment duration
There are multiple critical technical parameters that promote these biological responses. However, the most critical factors arguably are wavelength, energy density and duration of treatment (Figure 2). The wavelength is of particular importance, because it needs to match the absorbance spectrum of the desired photo-accepting molecule. The wavelength will also determine the penetration depth of the light being delivered (Figure 3), regardless of the device that produces the light energy.
The majority of wavelengths used clinically are between the red and near-infrared spectrum (600 to 1070 nm), which is also considered the “optical window” of light delivery, or the range of light where effective tissue penetration is maximized. Tissue chromophores, such as hemoglobin and melanin, have high absorption at wavelengths shorter than 600 nm, while at longer wavelengths water absorbs infrared light. This is why blue light (400 to 480 nm) is generally only used for superficial tissue treatments, and infrared light above 1100 nm is not used as frequently.
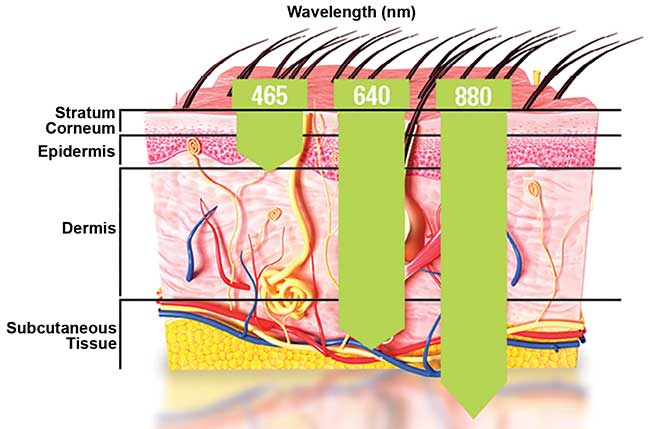
Figure 3. The wavelength of light used will determine the extent of penetration depth through the tissue. Human skin comprises multiple layers ranging from the superficial stratum corneum to the deeper subcutaneous tissue. Various wavelengths can be selected based on the desired target tissue and therapeutic outcome. Three commonly used wavelengths known to have different penetration depths through tissue are shown: 465 nm (blue), 640 nm (red) and 880 nm (near-infrared). Each wavelength is associated with particular beneficial effects, and the relative penetration depth through the tissue is depicted. Courtesy of BioPhotas Inc.
The energy density of the light used must be high enough to elicit the desired effect, but low enough not to induce toxic or adverse effects. Powers ranging from 1 to 1000 mW are commonly used. However, particular attention should be given to other optical parameters, which more specifically identify the dose of light being delivered (energy density, irradiation time, treatment interval and pulse structure). Generally, the clinical literature supports that treatments delivered multiple times a week over several weeks result in greater efficacy and clinical significance. However, these parameters will vary depending on the desired application.
Other additional considerations are the pulse frequency and the technological source of the light energy. Currently, there are no well-accepted or established effective pulse frequencies for given clinical applications. However, the pulsing of light is known to increase penetration depth, because high peak power is easily achievable even by using moderately energetic pulses. Pulsed light has been shown in some cases, such as wound healing and poststroke management, to have additional benefit in comparison to continuous light delivery.
Over the years there has been some contention as to whether the performance of incoherent light sources with wider bandwidth, such as LEDs, are comparable to coherent light sources with narrow bandwidth, such as lasers. However, when comparing laser and LED sources, both have shown similar clinical efficacy when optical parameters were closely matched. Consequently, evidence suggests that at the energy levels commonly used for LLLT, laser and LED sources could be used interchangeably and achieve similar if not identical efficacy. All of these factors should be carefully considered prior to selecting an appropriate light delivery device.
Selecting an LLLT device
It can be difficult, especially for new users of LLLT, to select a device that has been designed to address all of the key factors mentioned. There are numerous light sources available, ranging from more sophisticated advanced laser systems to basic home LED devices. More advanced laser systems tend to require more hardware components, offer additional tuning of optical parameters and are usually more suitable for advanced users who understand how to safely operate the device. Basic devices tend to feature LEDs, are intended to be operated by an individual with little or no experience, and pose no significant safety concerns.
Despite these generalizations, there will certainly be variation between different brands of devices and different types of devices. Consequently, the features required by each individual user will be highly dependent on their skill level in using such a system and, perhaps most importantly, the intended application.
Treatment area size and condition severity
Other considerations that must be made by the user of a LLLT device are the size of the treatment area and the severity of the condition. In some cases, either due to the nature of the treatment required or inability to access the target area, seeking a trained clinician is advised. Clinicians have access to devices such as treatment workstations that are not available to the general public and have the expertise to operate them safely. For general users who are looking for ease of use, devices that come preprogrammed with multiple operating modes for a number of clinical applications are ideal, such as BioPhotas’ Celluma. However, it is important to verify that the operating modes are consistent with the existing parameters demonstrated to be effective.
While lasers have traditionally been used for light therapy over the years, the use of LEDs has become increasingly popular for the treatment of skin diseases, wound healing and pain. Many LED arrays are now made to be easily portable, flexible and able to deliver light to a large treatment area. These characteristics can be very advantageous considering that it can be challenging to treat large areas of the body. The flexibility of some LED arrays allows them to adapt to the contours of the body and keep the source distance relatively constant (Figure 4). To maintain the desired treatment dose it is ideal that the distance between the source and the treatment area remain constant. This is important, because it is known that the intensity of light administered from a source decreases as the square of the distance from that source. However, each light delivery device will likely have some advantages and disadvantages depending on the desired application.
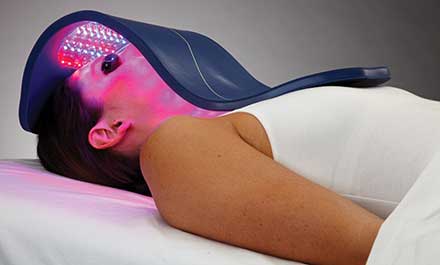
Figure 4. The distance between the light source and treatment area is a critical component of successful clinical outcomes. One method to improve even light dosemetry when treating a larger target tissue is to use a flexible LED array-type light source. The flexibility of the device allows for optimal fitting to the contours of the body. This allows for more even light delivery across a given location on the body, which represents a distinct advantage over similar, more rigid light delivery devices. Courtesy of BioPhotas Inc.
Growing acceptance
It is clear that there is a broad range of optical parameters reported to induce specific biological responses resulting in improved therapeutic outcomes. A critically important benefit of LLLT is the lack of significant side effects or adverse events associated with treatment, attesting to its safety. As evidence regarding the mechanisms and efficacy of this therapy increases, it is expected that the acceptance and widespread usage of LLLT will continue to grow.
Meet the author
Ryan Spitler is a postdoctoral research fellow at Stanford University in Stanford, Calif. He serves on the advisory board of BioPhotas Inc.; email: [email protected].