Miniature devices implanted in rodents have been effective for normalizing their heart rates through either electrical or optical stimulation.
IGOR R. EFIMOV, NORTHWESTERN UNIVERSITY, AND PHILIPP GUTRUF, UNIVERSITY OF ARIZONA
Heart disease is the leading cause of death in the developed world. Sudden cardiac death is triggered by the breakdown of a normal periodic heart rhythm, also known as the sinus rhythm. The emergence of aberrant, high-frequency sources of electrical activity in the heart causes cardiac arrhythmia and results in the failure of the heart to pump blood. While traditional methods, such as ablation and electrical stimulation or defibrillation, can efficiently treat this condition, they are painful and potentially damaging to the healthy myocardium and other tissues. Optogenetics has recently emerged as a potential approach to treating arrhythmias by light rather than by the electrical field. The miniaturization of delivery technology may soon provide lifesaving medical care.
Signals between cells
Multicellular biological organisms emerged from an initial evolution of signaling systems. This allowed two or more cells to communicate and synchronize their behavior and function to gain an advantage over their single-cell counterparts. Fundamentally, three signaling systems are known to biologists: chemical, mechanical, and electrical synapses.
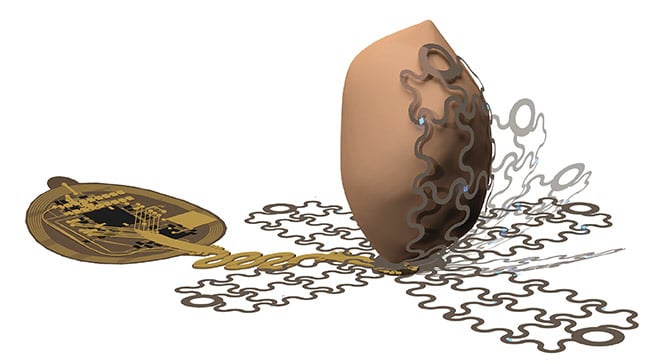
A photographic image of the cardiac interface before epicardial application. Courtesy of the Gutruf Lab.
The chemical synapse is the most ubiquitous and most studied signaling mechanism in the scientific community. It originates from the ability of a presynaptic cell to release a signaling molecule and then the ability of a postsynaptic cell to sense the molecule and transduce the detection into a response. A sophisticated multiprotein machinery has evolved to implement the functions of presynaptic signal emission via the production and/or sequestration of the signaling molecules, also known as messengers, and the release of the messengers upon receiving an appropriate upstream intra- or extracellular signal. Such messenger molecules include Ca2+ ions, ATP, IP3, PIP2, cyclic AMP, various hormones, neurotransmitters, and signaling peptides.
The mechanical synapse has evolved to transduce mechanical information between the cells. It is based on intracellular and extracellular multiprotein complexes and cellular receptors, including piezo 1 and 2, integrins, focal adhesion kinases, ankyrins, extracellular matrix proteins, and intracellular scaffolds. A mechanical synapse is a relatively low-frequency signaling system that provides mostly a proliferative response to pressure from intracellular organelles and proteins.
The electrical synapse achieves a higher speed and frequency than chemical or mechanical synapse communication. The electrical field and current flow are the foundation of this mode of intercellular communication. The electrical synapse is based on a sophisticated multiprotein complex machinery, which allows two neighboring cells to form a tight electromechanical junction capable of direct electric current flow and amplification between the two adjacent cells. This junction is a product of several families of proteins — including desmosomal proteins, connexins, and ion channels — which form a well-organized structure spanning across the membranes of the two communicating cells.
The majority of diagnostic and therapeutic interventions are based on accessing one of these signaling systems by biochemical, electrical, or mechanical sensing via cells or by stimulating cells within the body of a patient. Resuscitating a victim of sudden cardiac death is an example of such critical interaction with a dying heart by boosting its function using mechanical pumping, electrical synchronization, and pharmacological stimulation.
The role of optical signaling
Another signaling system is open to practitioners of diagnostics or therapeutics, particularly in the area of cardiology: optical communication. It evolved as a communication system between various organisms rather than within a single organism. Evolution has created numerous proteins and cellular processes capable of emitting or sensing light. Yet, until now, no cell-cell optical synapses have been discovered. The emerging field of optogenetics aims to close this gap and develop novel diagnostic and therapeutic approaches based on sensing light or light actuation.
Numerous efficient optical sensors and actuators have been developed in the last two decades, including optically controlled ion channels, receptors, and targeted proteins. In addition, numerous genetically encoded fluorescent reporters allow specific sensing of various physiological parameters and processes at the cellular level. The field of cellular biophotonics blossomed at the scientific bench and brought many new discoveries of fundamental biological mechanisms governing the functions of the nervous, cardiovascular, and metabolic systems1.
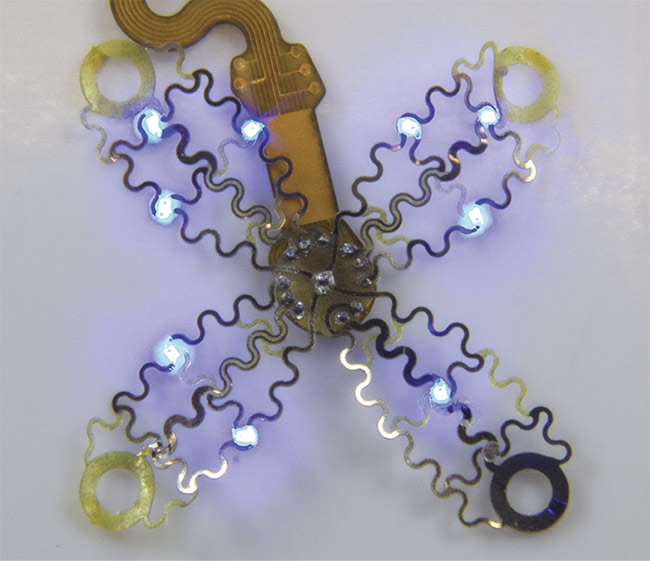
An exploded-view schematic of the optoelectronic device. Courtesy of the Gutruf Lab.
The translation of optogenetics to in vivo scientific and clinical applications, however, has been hampered by the lack of organ-conformal, fully implantable optoelectronics with footprint and operational lifetimes suitable for operation in humans. Most optogenetics research in rodents has required invasive exposure of the organ of interest, percutaneous light guides, or catheters, limiting the impact of the powerful technology. These interfaces typically rely on telecommunications-grade optical fibers that deliver light to the heart2,3.
While these invasive approaches have demonstrated the feasibility of optogenetic therapeutics in various animal models, they have had limited application beyond bench science because the animals were kept anesthetized during such acute experiments. A new therapy-delivery platform was needed to demonstrate the power of optogenetics in freely moving conscious animals with arrhythmia. Recent advancements in materials science — such as soft, stretchable, and flexible electronics with wireless power delivery — produced the technology for implantable organ-conformal optoelectronics coupled with optogenetics4.
Implantable optical devices
Based on this technology, a fully implantable multisite optoelectronic system has been developed that is capable of detecting and optically terminating an arrhythmia in rodents, either bradycardia or tachyarrhythmia5,6.
This new device class is enabled by soft-organ conformal optoelectronics, coupled with wireless power transfer that provides the energy required for in situ light generation, and ultimately senses electrical activity of the heart and produces optogenetic stimulation with high temporal and spatial precision when an arrhythmia is detected. This is similar to implantable cardiac pacemakers and defibrillators, which respond by electric therapy in response to bradycardia and tachycardia, respectively.
Wireless powering and soft mechanics are critical to enabling conformal cardiac interfaces that can deliver light on demand. Light delivery is accomplished via microscale light-emitting diodes (LEDs) that are directly integrated on soft, flexible substrates shaped to accommodate the strain of the beating heart while maintaining conformal contact. Existing pacing technology that uses single-use batteries cannot provide the energy needed for optoelectronic components in its current size. It would result in very bulky devices due to the increased demand.
The implementation of device-level soft mechanics also enables biointegration, resulting in fast recovery times and the distribution of electronics to the subcutaneous spaces. By comparison, current pacing technologies require invasive hooks to anchor electrodes in tissue. The new device architecture integrates advanced multimodal sensing abilities and rapid onboard computation using analysis software linked to the device to process diagnostics and interventions in real time. Fast response to arrhythmia is critical for lifesaving intervention in a clinical setting because the chances of successful resuscitation of a patient with ventricular fibrillation decline by 10% with every minute of lethal arrhythmia.
In this promising work, soft organ-conformal implantable optoelectronics are providing potential solutions in biomedical research. It is too early to predict how these techniques will impact the clinical workflow. Yet, it is clear that optogenetics as a whole will benefit from the improved temporal and spatial precision that is made possible by fully implantable miniature optoelectronic devices. Such devices may soon be able to control various physiological systems via optical sensing and stimulation of various proteins designed for the investigation of fundamental biology, disease pathogenesis, and possible future diagnostics therapies by light. Optogenetics is based on opsins, which are natural or artificial proteins that can be controlled by light emitted from an optoelectronic implant.
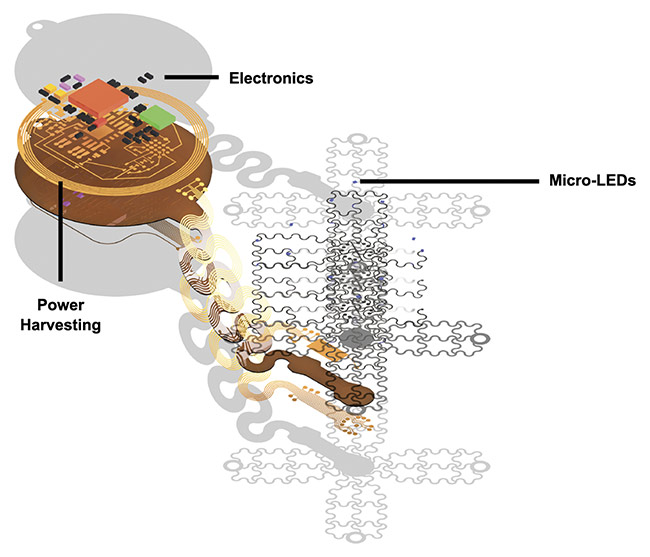
A rendering of the soft optoelectronic device applied en route to epicardial application. Courtesy of the Gutruf Lab.
Another important side to optoelectronic diagnostics and therapeutics exists, however. Numerous endogenous and artificial molecules possess fluorescent properties, and thus they can be used as light-based reporters of physiological and pathophysiological parameters of the body. Thus, combining optogenetics and fluorescence with optoelectronic implantable devices can provide a new, powerful approach to biophotonic theranostics or optotheranostics.
Moreover, optoelectronics could establish a bridge to the biological discovery of an optical synapse not yet developed through evolution or perhaps not yet discovered. If such an optical synapse does exist, the power of the presynaptic optical emitter must be matched to the sensitivity of the postsynaptic opsins. Demonstrating such optical cell-cell communication between two neighboring cells would require optoelectronic instrumentation of high fidelity, and this has not been developed yet. The work described has laid the foundation for the future development of implantable optoelectronics, which will help to discover and study optical signaling systems in vivo, if they exist, or to develop artificial optical signaling systems if they do not exist.
Meet the authors
Igor R. Efimov, Ph.D., is professor of biomedical engineering and medicine at Northwestern University. He is director of the Cardiovascular Engineering Laboratory, which focuses on the investigation of the mechanisms of cardiovascular disease, optical imaging of the heart, and the development of novel therapies; email: [email protected].
Philipp Gutruf, Ph.D., is assistant professor of biomedical engineering at the University of Arizona and is a Craig M. Berge Faculty Fellow. The Gutruf Lab focuses on creating devices that intimately integrate with biological systems through innovations in soft materials, photonics, and electronics to advance health diagnostics and therapeutics; email: [email protected].
References
1. E. Boyden et al. (2005). Millisecond-
timescale, genetically targeted optical control of neural activity. Nat Neurosci, Vol. 8,
pp. 1263-1268, www.doi.org/10.1038/nn1525.
2. T. Bruegmann et al. (2016). Optogenetic defibrillation terminates ventricular arrhythmia in mouse hearts and human simulations. J Clin Invest, Vol. 126, No. 10, pp. 3894-3904.
3. E.C.A. Nyns et al. (2017). Optogenetic termination of ventricular arrhythmias in the whole heart: towards biological cardiac rhythm management. Eur Heart J, Vol. 38, No. 27, pp. 2132-2136, www.doi.org/10.1093/eurheartj/ehw574.
4. M.K. Madrid et al. (2021). Advances in implantable optogenetic technology for cardiovascular research and medicine. Front Physiol, Vol. 12, p. 720190.
5. P. Gutruf et al. (2019). Wireless, battery-free, fully implantable multimodal and multisite pacemakers for applications in small animal models. Nat Commun,
Vol. 10, p. 5742.
6. J. Ausra et al. (2022). Wireless, fully implantable cardiac stimulation and recording with on-device computation for closed-loop pacing and defibrillation. Sci Adv, Vol. 8, No. 43, p. eabq7469, www.doi.org/10.1126/sciadv.abq7469.