Cornell’s greenhouse project delivers tons of hydroponic lettuce grown under a computerized lighting system.
Dr. Louis D. Albright, Cornell University
Imagine harvesting crops in New York state in the dead of winter. At Cornell University, a hydroponic greenhouse project is making that happen. In the controlled environment, it takes 35 days to grow one head of hydroponic lettuce, yielding a potential harvest of 390 tons an acre per year. That’s a lot more than the 45 tons of in-ground lettuce per acre per year grown in California and harvested from three crops. Central to the Cornell project is the greenhouse’s computerized lighting system.
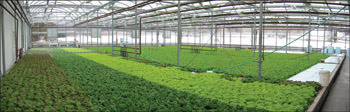
In one way, plants “see” the world much as humans do. That is, photosynthetically active radiation is located between 400 and 700 nm, which, for humans, is just inside the visible wavelength band. However, whereas the human eye is differentially sensitive to wavelength, plants perceive a photon at 400 nm much as they do a photon at 550 or 700 nm.
Specific wavelengths outside the photosynthetically active radiation band are important for morphological and flowering responses, but actual photosynthesis and growth are based on the number of photons (expressed as moles) received within the photosynthetically active radiation spectrum.
Two measures of light apply to plants: the photosynthetic photon flux density and the daily light integral. The former is the instantaneous photon flux of photosynthetically active radiation measured in μmol/m2s; to put this in perspective, full summer sunlight provides approximately 2000 μmol/m2s. The daily light integral, on the other hand, is measured in mol/m2 per day. Averaged over the Earth, it is approximately 26 mol/m2 per day. In the hydroponic greenhouse project, the outdoor solar daily light integral varied over the year from a low of 0.7 mol/m2 per day in December to a high of 64 in June, with a yearly average of 24.5 (Figure 1).
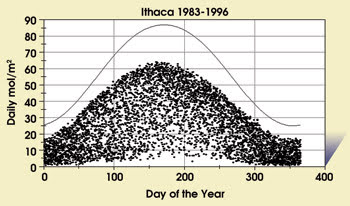
Figure 1. Fourteen years of natural light availability and variability are shown for Ithaca, N.Y., a climate with a cloudy winter. For comparison, the upper line is the calculated daily light integral outside the atmosphere on a plane parallel to the Earth’s surface. The ratios of actual data to this line are the averaged daily atmospheric transmittances.
Light is the only energy source available to plants for growth and development, yet it is the least adequately managed factor in the plant environment. Plants have evolved to survive with inadequate and highly variable light availability. They can do so even in high-latitude greenhouses during winter, but they do not thrive. They may not even produce a salable crop during the darkest weather.
Greenhouse operators are on an endless quest to produce the best possible crops at the lowest practical costs. To do this, the benefits of a mix of inputs must be balanced against their combined costs. Controlled-environment plant production under the same daily light integral and temperature is more likely to be uniform, consistent and of higher quality than is possible with traditional agriculture or with another type of greenhouse production. Supplemental lighting and movable shades are central to achieving consistent daily light integrals in many regions of the world; however, electricity for supplemental lighting can be expensive.
Supplementing CO2 increases light use efficacy and is typically less expensive to supplement than light in cold, cloudy weather. However, when ventilation exceeds some upper limit, supplementing CO2 becomes more expensive because of rapid losses out the greenhouse vents. Solving this optimization problem has been a long-term research objective of the controlled-environment agriculture program at Cornell.
At the university, research is under way to quantify the benefits of coordinating supplemental CO2 with assimilation lighting. Researchers also are seeking to define the relationships among length of day, the daily light integral and net productivity for a variety of crops, and to determine how controlling and managing the daily light integral and CO2 concentration can lead to optimum productivity. A third branch of research involves studying how plant growth and morphology can be manipulated using the spectral quality of incident light.
Light and shade
Plant responses to the daily light integral must be quantified to implement optimal control. An algorithm developed at Cornell in 1998 provides control of supplemental lighting and movable shades to achieve a consistent daily light integral. Called the Light and Shade Supplement Implementation, the algorithm showed comparable control accuracy when employed in a working greenhouse (Figure 2). Shade control, in addition to supplemental light control, is necessary for many crops. For example, if lettuce receives 10 to 20 percent too much light for two or three days in a row and grows too rapidly, it suffers a physiological disorder (“tip burn”) that renders it unsalable.
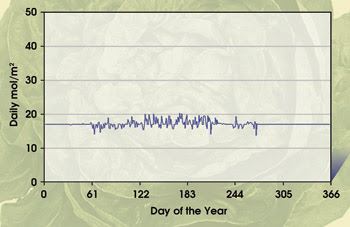
Figure 2. The simulated control adequacy of the Cornell University daily light integral control algorithm automatically controls light in the greenhouse.
Research at Cornell has led to a second computer algorithm, developed in 2007, which considers a range of light and CO2 control combinations for the following decision period. The algorithm, which estimates the ventilation rate expected and finds the optimum (lowest cost) combination, was tested by computer simulation for one year using hourly weather data. Results showed that coordinated control could save approximately one-half the electricity cost of lighting with no loss of lettuce production potential. Plans include testing additional crops, as funding for the project permits.
Although high-pressure sodium lighting generally is used in greenhouses because of its greater electrical efficacy, LEDs are of considerable interest as their efficacies improve. Plants have been grown to maturity using only 660-nm red LEDs, for example; however, not enough is known about commercial plant production using LEDs.
LEDs in greenhouses
A major research effort supported by the greenhouse industry in the Netherlands recently began exploring these issues. Current LED panel costs are too high for commercial adoption, and their electrical efficacies do not compete with those of high-pressure sodium lamps, but several companies are working to address these issues. When LEDs become practical, their ability to switch on and off without extended warm-up periods will render them of particular interest for providing supplemental light that optimizes lighting cost, as real-time electric rates vary by the hour during a day.
Supplemental lights for greenhouses (e.g., high-pressure sodium and metal halide) do not exhibit broad spectra of radiation. High-pressure sodium lighting provides limited blue light, for example. Published research has suggested that spinach growth can be accelerated by exposure to blue light at the beginning of the light period and to red light at the end. Adequate UV and blue light are thought to increase beneficial metabolites such as flavonoids, as well as anthocyanins for improved color and higher antioxidant content.
Many greenhouse coverings and, in particular, supplemental light sources significantly reduce the UV fraction of the total light received. Research and development opportunities exist to tailor supplemental light sources that provide more blue (and perhaps limited UV while being careful about health hazards for workers). A greater blue component in supplemental light also leads to compact plants, which may not affect human nutrition but may affect production and packaging practices.
Using plants for pharmaceuticals is another important area of future research, and light spectra can be used to effect metabolic processes and to enhance expression of the important chemical of interest. As a separate issue, limited research suggests that filtering specific wavelengths from light can disrupt the life cycles of certain greenhouse disease and pest organisms, reducing or eliminating the need for chemical control. Endless opportunities exist to improve plant lighting for objectives that go far beyond simply more growth.
Meet the author
Louis D. Albright is a professor in the biological and environmental engineering department at Cornell University; e-mail: [email protected].