Rajesh Patel, Scott White and Herman Chui, Spectra-Physics
A new laser system based on a fiber seed and a DPSS power amplifier produces UV and green wavelengths, allowing manufacturers to reduce costs while increasing volume and precision.
Over the past several decades, lasers have played an important role in the manufacture of advanced consumer electronic devices. From printed circuit boards to flat panel displays, hard disks to semiconductor wafers, laser processes are used to create the components of our smartphones, tablets, laptops and TVs. High-power, high-repetition-rate diode-pumped solid-state (DPSS) lasers, particularly at the ultraviolet wavelength, have proved themselves in a variety of cost-effective, high-speed micromachining processes that are key manufacturing steps for these ubiquitous electronic products.
Conventionally, Q-switched DPSS lasers operating in the nanosecond regime are used, although, more recently, both pulsed fiber and picosecond lasers have begun to appear on the manufacturing floor. These lasers, however, have characteristics that limit the extent to which process speed can be enhanced and manufacturing costs reduced.1 In particular, Q-switched DPSS lasers are constrained in their ability to scale throughput because the ability to increase pulse repetition frequencies (PRFs) is limited by changes in average power, pulse width and noise. Picosecond lasers deliver high-quality micromachining results, but throughputs are typically low – and costs high.2 Pulsed fiber lasers, on the other hand, have limitations in micromachining quality and throughput arising from low pulse energies, difficulties in achieving harmonics for UV and green wavelengths, and poorer beam quality.
As electronic devices continue to evolve, manufacturing processes also must advance. To meet the needs for higher-capability micromachining, the ideal laser could perform the processes carried out by today’s workhorse Q-switched DPSS lasers, but at higher PRFs. In addition, the high-precision manufacturing steps for today’s smaller and lighter mobile devices can begin to demand the quality and precision achieved by picosecond lasers, but cost-effectiveness must be maintained.
A new generation
A new high-power UV and green hybrid fiber laser simultaneously addresses micromachining throughput, quality and cost challenges. Achieving the goal of high power at high PRF, Quasar from Spectra-Physics outputs >40 W at 355 nm and >70 W at 532 nm, both at a PRF of 250 kHz. At the same time, it maintains the excellent beam quality and pulse-to-pulse energy stability, shorter pulse width and high pulse energy of DPSS lasers operating at more moderate PRFs.

Figure 1. Schematic diagram of Quasar laser technology. The system combines an advanced fiber seed laser with a DPSS power amplifier and adds harmonics technology.
Drawing upon the past decade’s advances in fiber lasers, the system uses a “best of both worlds” combination of fiber and DPSS technologies. As Figure 1 shows, it combines an advanced fiber seed laser with a DPSS power amplifier and adds field-proven, patented harmonics technologies. Targeting greater process control and higher micromachining quality and speed, it also features new TimeShift technology, which enables techniques such as pulse shaping, pulse splitting and burst mode operation. It also allows process engineers to dial in a wide range of software-adjustable pulse widths and pulse energies, independent of PRF, as well as offering the opportunity to create highly customized pulse-intensity waveforms in the time domain. This flexibility opens the door to achieving picosecondlike process quality while retaining the high throughput and cost-effectiveness of DPSS lasers.
Under the hood
Let’s look at some details. First, the new system produces the high pulse energies of today’s DPSS lasers: up to 200 µJ at 355 nm, more than enough for most precision micromachining processes. More importantly, high pulse energies can be delivered over a wide range of PRFs (Figure 2). At 300 kHz, for example, the UV laser puts out 39-W average power (pulse energies up to 130 µJ), allowing it to address most commercially valuable applications at very high speed.
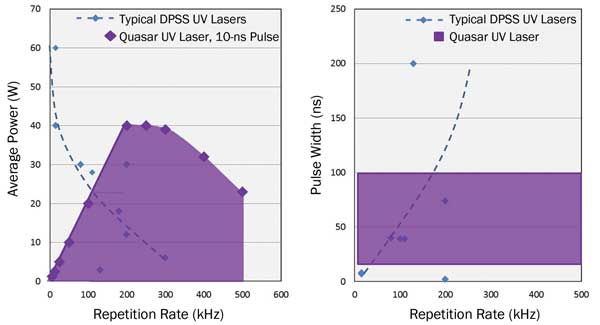
Figure 2. Comparison of UV output parameters between typical DPSS Q-switched lasers and the Quasar system.
When operating in the high-PRF regime of 250 kHz and above, the laser does not compromise on beam quality. TEM00 spatial mode (M2<1.3) is specified up to 400 kHz, ensuring that small focused spot sizes are maintained as process speed is scaled up. This is comparable to today’s best micromachining-suitable pulsed fiber lasers operating in the near IR. Unlike those lasers, however, the beam polarization state is linear – an important consideration for polarization-dependent downstream optics such as splitters, attenuators or modulators.
Pulse-width control
In many recently developed commercial micromachining applications, the effect of pulse width has become apparent even in the nanosecond regime.3 Although the physics differs from those of picosecond laser-material interactions, shorter nanosecond pulses move ablation results toward picosecondlike quality, with high peak power typically producing cleaner ablation features and a smaller heat-affected zone (HAZ). In contrast to picosecond performance, however, the per-pulse material removal rate in the short-nanosecond regime remains high.
Conventional Q-switched UV DPSS lasers can be designed to operate at 10 ns and below. However, this short pulse width is achieved only at low PRFs, limiting their usefulness for industrial applications that must be conducted at high speed to keep manufacturing costs low. For these lasers, as PRF increases, so does pulse width. In contrast, the new laser performs like other pulsed fiber lasers in this regard. Pulse width is decoupled from PRF, allowing the process engineer to throughput-scale previously developed applications with confidence. For example, let’s take an application currently in high-volume production that is performed with 10-ns, 100-µJ pulses at 150 kHz.
The new hybrid laser could PRF-scale the process to about 350 kHz, while maintaining pulse energy and pulse width at the previously defined conditions. Pulse energy and peak power at the high PRF is thus held at the same value that it has in today’s fully implemented 150-kHz process. Pulse-by-pulse process results are preserved, and to maintain the same pulse-to-pulse spot overlap, beam scanning speed is increased by a factor of 2.3.
As shown in Figure 3, not only can the new system deliver a constant pulse width as PRF increases (Figure 3a), but the TimeShift technology also enables the pulse width to be varied at fixed PRF (Figure 3b). This enables the process engineer to isolate the effect of pulse width on the ablation results; previously, using DPSS lasers, such a study was not possible without carrying out careful testing with multiple lasers. Further, the broad range of programmable pulse widths allows process developers to find their optimal value, yielding the best combination of ablation quality and material removal rate. Such process tuning is not at all possible with DPSS lasers.

Figure 3. Programmable pulse width: (a) 10-ns pulse width at 200 kHz and 500 kHz, (b) 10-ns and 50-ns pulse width at 300 kHz.
And TimeShift’s programmable pulse waveforms open the door to new techniques, where the ablation results may depend subtly on the details of the energy deposition rates in the workpiece. Pulse energies can be varied on a pulse-by-pulse basis, as shown in Figure 4a. For a particular workpiece material, perhaps the ablation rate or quality can be enhanced by hitting the material first with a high-energy pulse, followed by a smaller pulse to remove debris or otherwise clean up the ablation feature. Or perhaps it is better to preheat the material with a low-energy pulse and rapidly follow that with a high-energy pulse to achieve higher material removal rates.
If such subtleties aren’t needed, material removal rates can still be significantly enhanced by using TimeShift’s burst mode. By splitting the energy available in a single pulse into a number of shorter subpulses (Figure 4b), increased ablation rates are obtained without sacrificing micromachining quality.1 This is consistent with other studies finding enhanced material-removal rates for burst-mode ablation in the picosecond regime.4-5
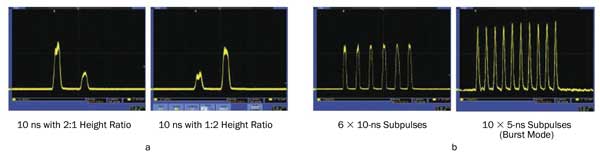
Figure 4. Programmable pulse waveforms: (a) pulse-by-pulse energy control; (b) pulse splitting.
Pulse splitting is quite flexible with this system. It permits tuning of the subpulse separation, the width of the subpulses, the number of pulses per burst, the amplitude of each subpulse within the burst, and the burst repetition frequency. The BRF can be varied through the full range over which the laser produces single (unsplit) pulses – i.e., 0 to 500 kHz.
Next, let’s briefly discuss how the new laser’s output characteristics can benefit two industrially significant laser micromachining processes. See Reference 1 for more detail.
Semiconductor fabrication
Laser scribing and dicing of silicon wafers has been in use for several years as an alternative to conventional dicing with a precision rotary saw. The laser process avoids the chipping and layer delamination, difficult or impossible to avoid with saws, that occurs when wafer thickness drops below about 100 µm or when fragile materials such as low-k dielectrics are present.
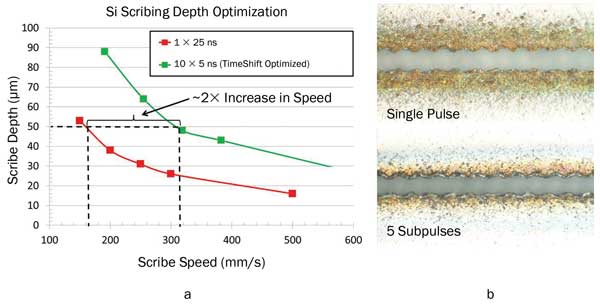
Figure 5. (a) throughput and (b) quality improvement in silicon scribing, achieved using TimeShift technology.
Recently, at Spectra-Physics’ applications lab, we investigated the scribing of 100-µm-thick semiconductor-quality silicon wafers with this system. Very high scribing speeds were achieved without thermal damage to the material. First, we established a baseline process using 25-ns pulses at 200 kHz (Figure 5a). We then scaled this process using TimeShift to create pulse bursts while maintaining constant 50-µm scribe depth. Bursts consisted of 10 subpulses of 5 ns, separated by 10 ns, and tests were conducted at a PRF of 255 kHz. As shown in Figure 5a, this resulted in an almost 100 percent increase in speed over the baseline process. Simply using the high-power and burst modes increased throughput by almost a factor of two. Further improvement may be possible using pulse-width optimization and pulse waveform shaping.
We also demonstrated that pulse splitting can improve micromachining quality. We observed the visual HAZ and debris zone surrounding the scribes when processed with both conventional single-pulse operation and 5-pulse burst mode. The burst-mode process produced smaller disturbed regions, as shown in Figure 5b. Moreover, with scanning speed held constant, a 25 percent increase in scribe depth was achieved by the burst-mode process.

Figure 6. Glass cutting at >1 m/s achieved using TimeShift technology. (a) Straight and curved line cut showing no chipping; (b) top and cross-section view of the cut showing smooth-cut edge and cross section.
Glass cutting for displays
In flat panel display manufacturing, cover glasses, touch panel glasses, and LCD and AMOLED modules are typically sized with a mechanical scribe-and-break process. This low-cost method generally works well for straight edges in nonhardened substrates. Current-generation mobile devices, however, use hardened cover glasses – and curves and nonlinear cutouts (holes and slots) are increasingly included in the product design. For these cases, next-generation laser processes show great potential. The challenge is to achieve high-quality cuts and high throughput while avoiding yield losses from chipping and microcracking.
In our preliminary applications lab studies, using a patent-pending process, the Quasar 355-40 has demonstrated significant advances in glass cutting over traditional laser machining processes. The control of pulse temporal characteristics made possible by TimeShift technology has enabled high-quality cuts at high speed. Figure 6 shows results achieved in 0.55-mm cover glass at >1 m/s linear cutting speed of straight and curved lines. Minimal chipping (<10 µm) was observed – and edge roughness was minimal and well within the acceptable range of typical manufacturing requirements.
Meet the authors
Rajesh Patel is director of strategic marketing at Spectra-Physics in Santa Clara, Calif.; email: [email protected]. Scott White is senior manager of product marketing at Spectra-Physics; email: [email protected]. Herman Chui is the company’s senior director of productmarketing; email [email protected].
References
1. R. Patel and J. Bovatsek (March 15, 2013). High speed micromachining with high power UV laser. Proc SPIE, Laser-based Micro- and Nanopackaging and Assembly VII, Vol. 8608, http://proceedings.spiedigitallibrary.org/proceeding.aspx?articleid=169011.
2. J. Sercel et al (October 2012). Ultrafast lasers: picosecond laser micromachining produces high-quality results. Laser Focus World, Vol. 48, No. 10, pp. 57-60.
3. R. Patel et al (January 2010). Why pulse duration matters in photovoltaics. Las Tech J, Vol. 7, No. 1, pp. 21-24.
4. W. Hu et al (February 2010). Modeling of multi-burst mode pico-second laser ablation for improved material removal rate. Appl Phys A, Vol. 98, Issue 2, pp. 407-415.
5. R. Knappe et al (2010). Scaling ablation rates for picosecond lasers using burst micromachining. Proc SPIE, Laser-based Micro- and Nanopackaging and Assembly IV, Vol. 7585, http://proceedings.spiedigital library.org/proceeding.aspx?articleid=1337000.