These low-light imaging detectors offer high sensitivity at near-IR wavelengths.
Ravi Guntupalli, Princeton Instruments
Thanks to advanced sensor and camera technologies, scientific low-light imaging detectors now boast more than 90 percent quantum efficiency, <1 electron root-mean-square read noise and negligible dark current. Although standard and electron-multiplying CCD cameras perform well in the ultraviolet-to-visible region, they pose unique limitations in the near-infrared (700 to 1100 nm). The availability of cooled deep-depletion CCD cameras is filling the gap and enabling scientific research, development and process control fields in such disparate applications as solar technology, astronomy and Bose-Einstein condensate.
In solar technology, deep-depletion cameras are used to determine the efficiency of solar cells. Researchers can use imaging techniques such as photo- and electroluminescence to quantitatively measure solar cell efficiency. These methods spatially map the efficiency by means of the images captured after the cell has been excited by a light source or an applied bias voltage. For these applications, standard front-illuminated CCDs fall short of detecting the lowest levels of luminescence, especially in the 1000- to 1100-nm region. Although indium-gallium-arsenide detectors do provide sensitivity beyond 1000 nm, they suffer from lower spatial resolution and higher dark noise.
Near-IR imaging is of interest to solar astronomers. For example, a group from the University of Hawaii at Manoa in Honolulu captured images in the near-IR spectrum of the sun’s outer corona — a region visible only during a solar eclipse. The scientists used multiple cooled deep-depletion PIXIS: 1024BR detectors from Princeton Instruments of Trenton, N.J., to capture the imagery. Upon examining it, they concluded that there is a surprising amount of gas in the corona and that it is hundreds of degrees cooler. Researchers selected the deep-depletion cameras for their quantum efficiency in the 780- to 1100-nm region, for their low dark current and for their negligible etaloning — a phenomenon that occurs when the incident light creates constructive and destructive interference in the detector.
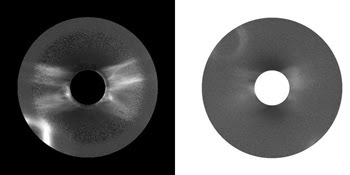
Figure 1. Near-IR images of the sun’s outer corona were captured during a solar eclipse. The highly sensitive near-IR instruments used bandpass filters centered at 789.2 nm (left) and at 1074.7 nm (right) in front of back-illuminated, deep-depletion PIXIS: 1024BR cameras. Images courtesy of Shadia Habbal, University of Hawaii.
In Bose-Einstein condensate applications, investigators use absorption and phase contrast imaging in the near-IR region to visualize — and extract useful information about — the cloud of atoms. This cloud forms when matter made from matter waves, which are formed when a gas composed of a certain kind of particles — referred to as “bosonic” — is cooled to below a critical temperature, typically only a few nano-kelvins. In this environment, the matter wavelength becomes so large that the wavelike atoms overlap and start to oscillate in concert, forming the cloudy condensate.
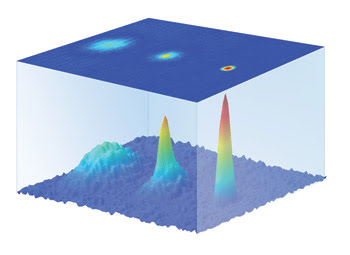
Figure 2. Phase contrast images of Bose-Einstein condensate of rubidium atoms were captured using a deep-depletion CCD camera (shown in 3-D pseudocolor). Data courtesy of Brian P. Anderson, University of Arizona in Tucson.
High sensitivity
Silicon-based detectors such as CCDs can detect a wide range of wavelengths, from deep-UV to near-IR. Three types of CCD technologies are used widely for scientific imaging: front-illuminated, back-illuminated and back-illuminated deep depletion.
In front-illuminated technology, the light enters the detector through polysilicon gates and is absorbed in the depletion region of the silicon. Because a fraction of incoming light is lost at the gates, the detectors offer only moderate quantum efficiency. (Quantum efficiency is the fraction of incoming photons absorbed by the silicon.) In back-illuminated and back-illuminated deep-depletion detectors, however, the light enters directly into the silicon. As a result, these devices offer maximum sensitivity over a wide range of wavelengths. Especially, the deep-depletion detectors, with a thicker silicon or depletion zone, allow less-energetic near-IR photons to be absorbed more efficiently. Consequently, they offer the highest near-IR sensitivity (Figure 3).
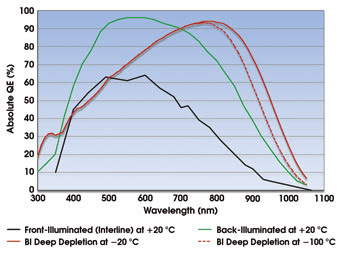
Figure 3. Back-illuminated deep-depletion CCDs offer the highest sensitivity in the near-IR region, even when they are cooled to –100 °C.
It is to be noted that the near-IR quantum efficiency of most detectors is reduced with lowering of detector temperature.
In Figure 3, the quantum efficiency of typical deep-depletion back-illuminated CCDs is plotted at two temperatures:
–20 and –100 °C. From the graph, it is clear that deep-depletion devices offer superior sensitivity to that of front-illuminated and standard back-illuminated devices at any operating temperature.
The slight reduction in quantum efficiency must be evaluated against significant decreases in dark current and in overall detector system noise. The dark current reduction is several orders of magnitude from that of uncooled detectors. For optimum performance, cameras such as the PIXIS offer precise temperature control in the range from room temperature all the way down to –100 °C.
Another important factor to consider in near-IR applications is etaloning, which is most visible in standard back-illuminated CCDs; however, deep-depletion detectors effectively eliminate it by moving the standing wave beyond the range of silicon detection.
In addition to sensitivity, users also must take into account pixel format and CCD sizes to best match the object size. Cooled deep-depletion CCD cameras are expanding the frontiers of low-light near-IR imaging below 1100 nm and are expected to remain a valuable tool for fun?damental research or process control.
Meet the author
Ravi Guntupalli is the business manager at Princeton Instruments in Trenton, N.J.; e-mail: [email protected].