Researchers are targeting a comprehensive understanding of lymphatic vessels with potential implications for improved treatment.
MARCO ARRIGONI, COHERENT INC.
The lymphatic system is an underinvestigated part of the vascular system. A team led by Friedemann Kiefer at the European Institute for Molecular Imaging (EIMI) at the University of Münster in Germany is aiming to change that in ongoing studies of the formation and function of lymphatic vessels in lab mice.
The researchers use an arsenal of fluorescent microscopy techniques to probe these vessels and unravel the genetic control of formation, function, and repair. Multiphoton microscopy, often using triple-transgenic mice, is proving to be a critical tool in these studies because it is the only high-resolution method that can image at subcellular resolution deep within live and in vitro tissue samples.
The circulatory system in mammals, and most importantly in humans, has been exhaustively studied and characterized. Yet the lymphatic system remains poorly understood at many levels. In major surgery, this limited understanding means lymphatic vessels are routinely excised or damaged inadvertently. This surgical damage is a leading cause of lymphedema, in which a patient’s limb can painfully swell, become fibrotic, and lose function.
Normal function of pressurized blood flow means that the fluid component of blood (plasma) leaks out of the capillaries in every tissue. The plasma is collected in tiny blind vessels that are connected to larger collection vessels. Now called lymph, the fluid flows through nodes in this system of vessels where the fluid is screened for pathogens and where cancer and infections often manifest as a result.
Valves in the vessels ensure unidirectional flow, and movement is driven by smooth-muscle contraction in the larger vessel walls and secondary pressure from nearby arterial pumping. The lymph then re-enters the arterial-venal system at two interconnections below the clavicles (collar bones). Even larger lymph vessels may be in a collapsed state, which, together with the fact that lymph is a clear liquid, explains why lymph vessels are often unseen and thus damaged during surgeries.
“We are trying to fill in the huge gaps in the understanding of the lymph system,” Keifer said. “This includes morphogenesis — how the vessels are first created in an embryo — to normal function at the cellular level; the consequences of hypoxia, or depleted oxygen; and how the development of both lymph and blood vessels are affected by tumors, strokes, arteriosclerosis, trauma, and aging. It’s basic biological research, but with obvious potential for improvements in medical treatments and outcomes.”
In a recent landmark publication1, the researchers first revealed how mammalian (murine) lymph vessels are derived from venous endothelial cells during embryo development. Surprisingly, they found that the process is initiated by delamination and cell migration from the cardinal vein, rather than from sprouting, which might be expected intuitively.
In major surgery, the limited understanding of the lymphatic system means that vessels are routinely excised or damaged inadvertently.
Kiefer’s group uses several fluorescence microscopy techniques in its studies. These include conventional wide-field epifluorescence microscopy, confocal microscopy, 3D reconstruction microscopy, and selective plane illumination-based ultramicroscopy (often called light-sheet microscopy). All of these play valuable roles in imaging tissue samples and early-stage murine (mouse) embryos. But none of them have the ability to image within a live animal or thick tissue sample.
For deeper images, the group exclusively uses multiphoton excitation: specifically, two-photon laser scanning microscopy (2P-LSM). As an excitation source, they use either a tunable titanium:sapphire (Ti:sapphire) laser (Coherent Chameleon) or one of the latest ytterbium-fiber-based (Yb-fiber) tunable one-box laser sources (Coherent Discovery) (Figure 1).
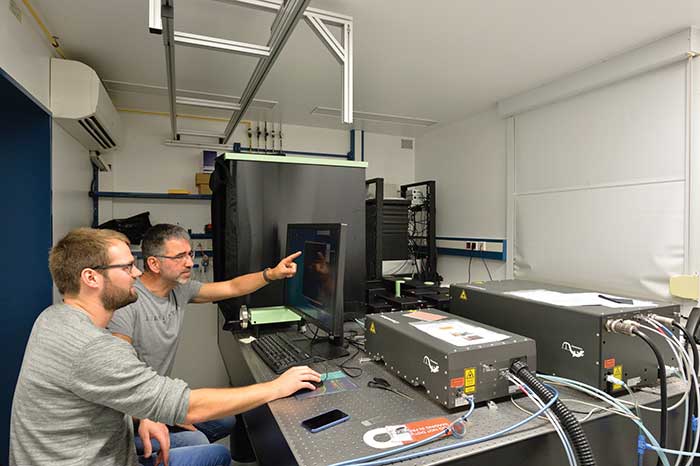
Figure 1. Kiefer team members Nils Kirschnick (left) and Michael Kuhlmann at the multiphoton microscope equipped with both a titanium:sapphire laser (Chameleon Vision) (closest to men) and one of the next-generation lasers based on ytterbium fiber (Chameleon Discovery) (far right). Courtesy of Kiefer group/EIMI Münster.
The researchers need deep-imaging capability for many of their studies. “With early-stage embryos, some of the developing vessels are almost at the surface,” Keifer said. “But when we look at the effects of strokes or arteriosclerosis in adult mice through a cranial window or tumors through a skinfold cavity, or even tumor tissue samples from biopsies, we sometimes need a penetration depth of hundreds of microns.”
Two- and three-photon excitation of fluorescence using a tightly focused NIR ultrafast laser presents several advantages. Fluorescence is only excited at the narrow beam waist, delivering 3D resolution and eliminating out-of-focus background noise. In addition, compared to visible wavelengths, attenuation of the laser beam is much lower for the NIR wavelengths needed for two-photon excitation, enabling deeper penetration. Just as important, live samples can be subjected to prolonged and repeated exposure with minimized photodamage because most of the NIR laser light is not absorbed.
High power allows deeper imaging, which is why the newest laser in the Kiefer lab is of the Yb-fiber type; it produces higher power at wavelengths well beyond 1 µm and supports the imaging of long-wavelength fluorophores at greater depth. A carotid artery example (Figure 2) from the group’s parallel studies of blood vessels dramatically demonstrates this deep-imaging capability.
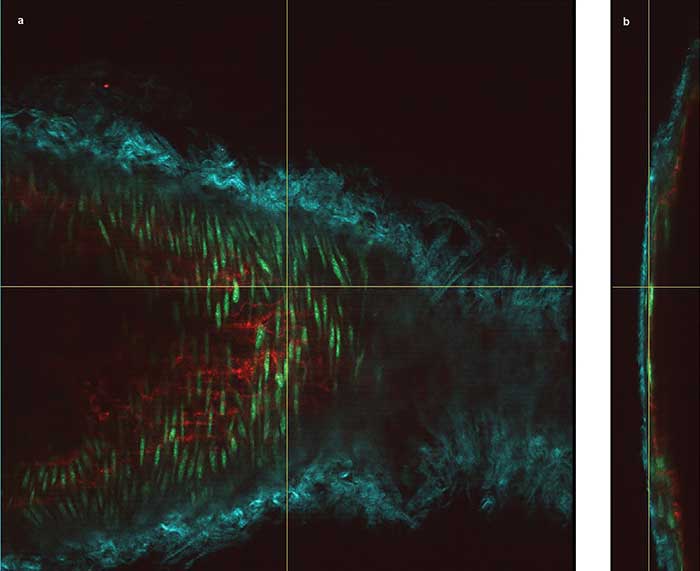
Figure 2. The vessel wall of a mouse carotid artery, showing en face (a) and orthogonal views (b) obtained through deep imaging. The cyan structures are collagen fibers (via second-harmonic generation detection), the red are endothelial cell interfaces labeled with Alexa 647, and the green shows nuclei of the smooth muscle cells labeled with Cyto41. Courtesy of Dominic Depke/EIMI Münster.
The specimen was excited at 830 nm and 1100 nm simultaneously. The cyan structures are collagen fibers — using second-harmonic generation (SHG) excited at 1100 nm — around the arterial vessel, together with smooth muscle cells of the media (green nuclei stained by Cyto41). Similar to all blood vessels, the innermost lining of the carotid artery is formed by a single-cell layer of endothelial cells, which were stained here with an Alexa 647-coupled PECAM-1 antibody (red). The red staining indicates the interendothelial cell-cell junctions.
“The carotid wall that is imaged in this maximum-intensity projection corresponds to approximately 80 µm,” Kiefer said. “With normal epifluorescence or even confocal microscopy, it is absolutely unthinkable to even penetrate the collagen fibers of the elastic layer of a large arterial vessel. Here, we go through the elastic fibers, then the smooth muscle layer underneath all the way to the lining endothelium. In the 3D rendering, we basically show a slanted cut through the vessel and include only a very few layers in this image. Otherwise, the highly detailed picture becomes so congested, people might not recognize any of the structures we are talking about.”

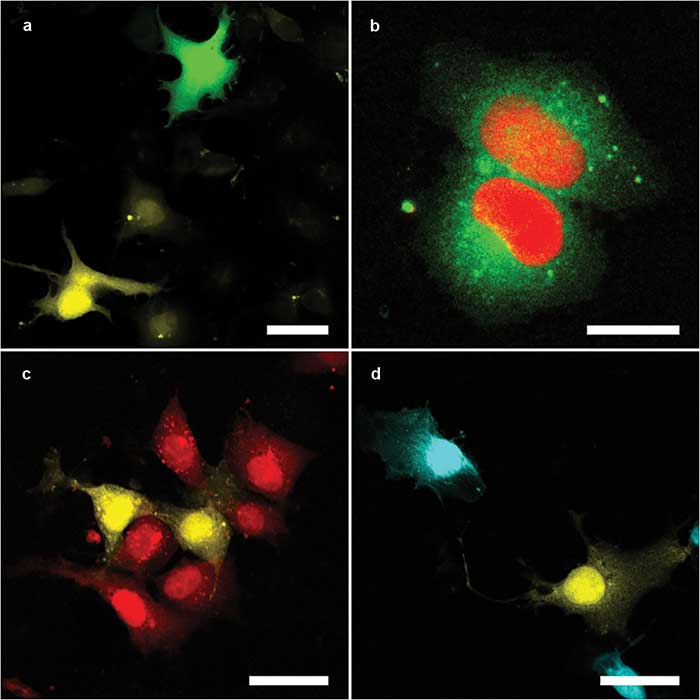
Figure 3. The multicolor capacity of the Kiefer lab setup. Mixtures either of Cos1 cells expressing different fluorescent proteins — enhanced green florescent protein (eGFP) (green) and mOrange2 (yellow) (a); mOrange2 (yellow) and mCherry (red) (c); and mOrange2 (yellow) and mPlum (cyan) (d) — or a single Cos1 cell expressing two fluorescent proteins in different compartments — mClover3 (cytoplasmic, green) and mRuby3 (nuclear, red) (b). Panels a and b were excited with 820-nm (eGFP, mClover3) and 1100-nm (mFruit, mRuby3); panels c and d with 1100-nm (mFruit) only. Scale bars = 20 µm. Courtesy Nils of Kirschnick and Abel Pereira da Graca/EIMI Münster.
Another reason for choosing the Yb-fiber-based laser was its broad tuning range and fast tuning speed. These features are required because the researchers typically want to image two different fluorescent proteins (and often a third fluorescent dye) near simultaneously, where these fluorophores are chosen to have well-separated excitation/emission spectra to maximize contrast. They also sometimes detect SHG signals, which can image collagen fibers without any staining.
This fast multiwavelength capability is needed to take full advantage of the rare (and valuable) triple-transgenic mice bred by the researchers.
Live samples can be subjected to prolonged and repeated exposure with minimized photodamage because most of the NIR laser light is not absorbed.
“Using established protocols, an mFruit gene such as mOrange2 is linked to a promoter gene for lymphatic vessel proteins,” Kiefer said. “However, there is no single protein target that is a unique marker for lymph vessels, so we have to use a combination of two proteins, like mOrange2 and tdTomato. And we often use even a third marker, for example UnaG (a protein derived from Japanese eel), which fluoresces when it binds to bilirubin and allows us to map hypoxia.”
In addition to fluorescent labeling, the team also breeds mice with individual genes selectively deleted, which is essential for some of the studies in embryonic development of blood and lymph vessels and also in disease models. For example, they use mice lacking the apolipoprotein E (ApoE) gene, which causes them to have high plasma serum cholesterol levels and to develop spontaneous arteriosclerotic lesions.
Kiefer stresses that all the animals are humanely treated according to the legal regulations of the German Animal Welfare Act.
Some recent images acquired with 2P-LSM from the ongoing study show the structure of lymphatic vessels in different organs (Figure 4). Specifically, these images from the diaphragm and mesentery of a mouse show lymphatic vessels that are unmistakably identified by the fluorescent red and orange proteins they specifically express, with no additional staining procedure.
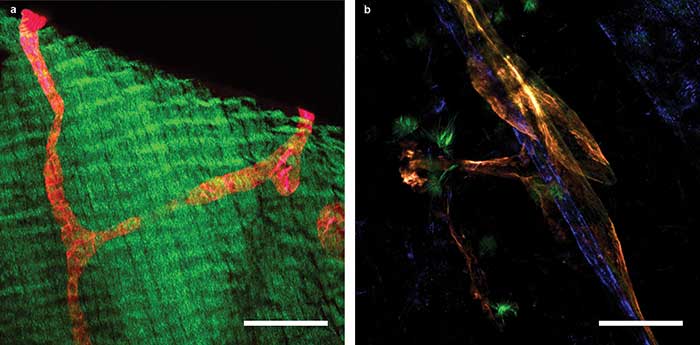
Figure 4. 2P-LSM images of a transgenic mouse expressing the tdTomato protein under the control of a promoter that drives expression predominantly in lymphatic vessels. A lymphatic collector vessel (red) within the muscle of the diaphragm; green fluorescence is generated by SHG of muscle fibers (a). Mesenterial lymph (orange) and blood (blue) vessels (b). Scale bars = 100 µm. Courtesy of Nils Kirschnick/EIMI Münster.
The lymphatic system is poorly understood at virtually every level, yet its disruption by disease and surgical trauma has functional and painful consequences for many people. Multiphoton microscopy is proving to be a powerful tool for researchers to shed light on the exact mechanisms by which this occurs.
Meet the author
Marco Arrigoni is a director of strategic marketing at Coherent Inc. He covers the scientific research markets; email: [email protected].
Reference
1. Hägerling et al. (2013). A novel multistep mechanism for initial lymphangiogenesis in mouse embryos based on ultramicroscopy. EMBO J, Vol. 32, Issue 5, pp. 629-644.
Minimizing Laser Downtime
In many of Friedemann Kiefer’s experiments, it is necessary to repeatedly image the same sample at different intervals over a period ranging from a few hours to several months. This requires long-term laser stability and high uptime.
“Even in a small animal like a mouse, arteriosclerosis develops over weeks,” he said. “And some tumors develop over an even longer time frame. In a worst-case scenario, if we lose the ability to image in a certain time window, then the entire experiment is lost, often together with a valuable triple-transgenic mouse, which we have to euthanize.”
In next-generation lasers such as the one Kiefer used, laser manufacturers maximize data throughput and minimize unscheduled downtime in two ways.
First, the laser is designed to deliver industrial reliability by being tested to destruction multiple times during design and prototyping using rigorous highly accelerated life test (HALT) protocols. In production, it is tested beyond its performance specifications before shipping from the factory using highly accelerated stress screening (HASS) methods.
Second, the laser’s onboard smart self-diagnostics and control systems support remote service via an internet link, rather than waiting a week or two for a service technician to visit.
To date, Kiefer’s group has had three minor issues with reduced output power. In each case, laser performance was restored to specification the next day, preventing any loss of experiment.