After displacing conventional lasers in the factory, they target new applications.
Robert Afzal and Andrew Brown, Aculight Corp.
Fiber lasers, once laboratory curiosities, evolved first into the workhorses of telecommunications. In their second transformation, they found work in a host of marking, welding and cutting applications on the factory floor.
Today the versatile instruments are proving their worth in a range of established and emerging applications, from medical procedures to directed-energy weapons systems to airborne active imaging platforms.
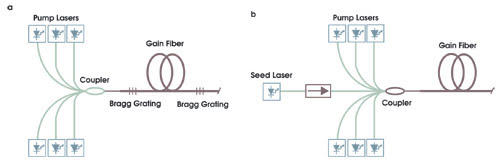
The intrinsic geometry of fiber lasers (a) offers a compact, robust source with high power, excellent pulse stability and conversion efficiency up to 30 percent. Incorporated with a MOPA configuration (b), properties such as pulse duration, peak power, spectral content, polarization and beam quality can be controlled easily.
Among the factors driving this evolution is the fiber laser’s ever-growing output power – from tens of watts a decade ago to several kilowatts today. Other key factors include their high-quality beams, electrical efficiency, and compact size and ruggedness. Lastly, their potential for eye-safe operation at high repetition rates makes them particularly good candidates for active imaging.
Limits? Yes.
Fiber lasers have their limitations, however. Nonlinear processes intrinsic to the fiber limit their ability to store energy and generate significant amounts of peak power. So it is unlikely that they will ever compete with diode-pumped solid-state (DPSS) lasers for applications requiring pulses of 100 mJ or greater.
A fundamental issue with many conventional DPSS lasers is free-space optics and efficient removal of waste heat generated by the lasing process, which limits their power and beam quality. Fiber lasers neatly finesse this issue with their all-in-fiber architectures and huge ratio of surface area to volume.
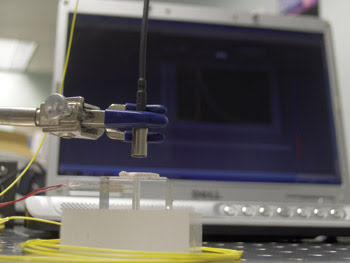
Researchers at Beckman Laser Institute, University of California, Irvine, investigate the use of a mid-IR fiber laser for surgery of the bones in the ear. The picture shows a thermocouple measuring the temperature in an inner-ear model while a bone is irradiated with a fiber laser. Fiber lasers also are used to stimulate nerves in neurophysiological studies.
But this finesse comes at a cost: High optical intensities, propagating over long lengths of fiber, can trigger nonlinear processes that drain the laser’s power. Large-mode-area fibers have helped alleviate the problem but, again, at a cost. Higher-order transverse modes can propagate in larger waveguides, which may affect beam quality adversely. Techniques such as careful injection of pump photons and of bending the fiber can reduce these problems but not always eliminate them.
DPSS lasers first enabled airborne active imaging applications, but these sources tend to be large and to require significant cooling. They also most often operate at 1μm, a wavelength that is not eye-safe.
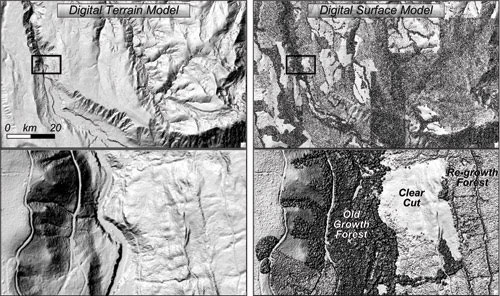
These shaded relief images show the west flank of Mount Rainier, Wash. Compiled from data collected using an airborne laser imaging system, they reflect differences in the “first” and “last” returns of each laser signal. On their journey toward the ground, laser pulses first encounter the reflective surface of higher surfaces, such as a forest canopy or the top of a building. Data from the pulses (right) can distinguish among various types of land cover. The left images were compiled from pulse data that reached the ground and, thus took longer to return to the detector. Last returns enable construction of topographical models of the Earth with vegetation cover and buildings removed. Images on the bottom, with 1.8-m spatial resolution, reflect the areas highlighted by the boxes in the upper images. All images courtesy of David Harding, NASA Goddard Space Flight Center.
Fiber lasers offer a comparatively efficient, rugged and compact alternative that also can help improve the performance of conventional active imaging designs. The 1-μm output of Yb-doped fiber lasers offers a drop-in replacement for legacy imaging systems based on Nd:YAG and other solid-state lasers. Alternatively, erbium-doped fibers operating in the eye-safe regime of 1.5 μm could help spawn new applications. Such devices are finding increasing utility in lidar systems aboard low-flying platforms, in machine vision and on robots and unmanned vehicles.
That isn’t to say fiber lasers will displace DPSS lasers entirely. Flash lidar used for target identification, for example, requires high-intensity pulses more characteristic of the Nd:YAG lasers. But in the commercial sector, fiber lasers are enabling next-generation scanning lidar systems that can deliver three times greater precision and 10 to 100 times greater data density. These improvements derive from the comparatively shorter pulse widths and faster repetition rates that compact fiber lasers allow. Plus, when configured in a master oscillator power amplifier (MOPA) construction, fiber lasers offer much more access and control over pulse properties on the fly.
Put simply, a lidar system fires a pulse of laser light at the ground and measures how long it takes to return. The clock stops when the peak energy of the emitted pulse returns to the detector. So, shorter pulses offer less ambiguity when measuring return time.
The solid-state lasers in conventional active imaging systems emit pulses about 10 ns long. Measured in spatial terms, that pulse would be about 3 m long. By comparison, the 2-ns pulse widths possible from fibers lasers emit pulses less than 1 m in length that, in pragmatic terms, mean less ambiguity – i.e., higher precision – when calculating return time.
Better Resolution
Also, the faster a laser’s repetition rate, the more spots it can put on the ground in a given time span. Where conventional pulsed solid-state lasers average about 10 kHz, fiber lasers offer repetition rates ranging from 20 to 30 kHz to several megahertz while maintaining peak powers in excess of 10 kW. The result is images with greater spatial resolution.
In practical terms, these performance properties of MOPA-configured fiber lasers enable pilots to collect data of comparable quality while flying higher – reducing flight time and associated costs of the data set – or to collect far more data when flying at comparable altitudes.
The market is driven by demand for geospatial data, generally in the form of high-resolution, three-dimensional, geolocated images used for civil engineering, urban planning, disaster preparedness and other purposes. More advanced applications appear in tactical military systems and prototypes, including autonomous vehicles requiring machine vision for collision avoidance.
Incorporated into a MOPA architecture, fiber systems offer flexibility in design and performance to match ultimate end-user system requirements. But it would be an oversimplification to suggest that you could simply load a marking laser onto an active imaging platform. Integrity of the data is of utmost importance in these systems, and virtually every application poses its own peculiar demands on the design of the laser and on its output profile.
The compact footprint, low maintenance and power-efficient operation make fiber lasers well suited for medical applications in the office or clinic. Moreover, the consistent beam quality and flexibility of fiber lasers make them attractive medical tools.
Previously, dermatologists used CO2, dye or solid-state lasers for bulk treatment of the skin – essentially ablating layers of skin – often beginning with the outermost layer. Erbium-doped fiber lasers emitting narrowly focused 1.5-μm beams allow deeper penetration of the epidermis that, in turn, enable development of pinpoint treatments based on a technique called fractional photothermolysis.
This technique uses a Gaussian-profile beam to form thousands of microthermal zones hundreds of microns below the surface of the skin and about 100 μm wide. Unlike bulk ablation, this preserves the outermost layer of the skin and stimulates new collagen growth.
Thulium-doped fiber lasers also are gaining interest as surgical tools because of their operating wavelengths in the water-absorption region, between 1.8 and 2.1 μm. They offer a comparatively low-cost, high-precision alternative to the holmium and frequency-doubled solid-state lasers that commonly target these applications.
The short-pulse, high-peak-power and broad-bandwidth capabilities of fiber lasers also are driving developments in medical imaging modalities, such as optical coherence tomography (OCT).
Increased bandwidth is important because it translates into decreased coherence length, and that increases the resolution of the OCT image. Modifying the seed linewidth or fiber nonlinearities can minimize the coherence length of a fiber laser beam to deliver OCT images with axial resolutions as low as 5 μm.
The cutting edge
The military has great interest in directed energy weapons, such as the U.S. Air Force’s Airborne Laser System (ABL), and fiber lasers have been added to its list of candidate sources for future systems. The ABL is intended to provide a speed-of-light defense against missiles, but similar systems are being researched for more tactical military applications, including littoral warfare and antiaircraft defenses.
Unlike chemical lasers – such as that in development for the ABL – fiber lasers require only an electrical source to operate. Their diodes are compact, generate no chemical-reactant waste and, most importantly, are highly efficient at converting electrons to photons to generate a high beam quality. Lastly, their efficient operation minimizes thermal loading of the glass fiber, enabling up to kilowatts of output power.
Although such outputs are impressive on the factory floor, directed energy applications generally require tens to hundreds of kilowatts. This goal is entirely possible by combining the beams of several fiber lasers.
Coherent and spectral beam-combining experiments are being pursued actively to gang the outputs from many lower-powered fiber sources to generate a single, high-power beam that still maintains many of the key advantages of a single fiber source.
Coherent beam combining is similar in technique to the phased array in some radar systems, albeit at significantly reduced wavelengths. Spectral beam combining is a power-scalable technique that overlays the wavelength-offset outputs of many laser emitters into a single, near-diffraction-limited beam.
At Aculight, we have used this technique to achieve the high-density combining of 1400 individual semiconductor lasers into a single beam. When we applied the same approach to fiber lasers, we demonstrated power scaling of multiple fibers into a diffraction-limited beam of more than 500 W.
Other emerging fiber laser applications promise to arise from their facility for nonlinear wavelength conversion. One is a green laser, operating at 532 nm, for underwater communications. Taking advantage of telecommunications technology, Aculight demonstrated such a source, based on a directly modulated 1064-nm semiconductor laser amplified in a multistage ytterbium-doped fiber amplifier. The light was then frequency-doubled with an LBO crystal. This approach enabled us to generate more than 50 W of green output power from a single-fiber MOPA.
We also have efficiently down-converted, in an optical parametric oscillator, a single-frequency 1-μm fiber laser into the mid-IR, generating tunable, single-frequency light with several watts of power. This was once the domain of finicky color-center lasers and lead-salt diodes capable of generating only milliwatts. Applications for this product are as diverse as high-resolution spectroscopy, atom trapping, remote sensing and defense of aircraft against heat-seeking missiles.
Meet the authors
Robert Afzal is the vice president of research and development at Aculight Corp; e-mail: [email protected].
Andrew Brown is senior director of R&D for Global Business at SPIE.SPIE.org
How it Works
In its simplest form, a fiber laser can be viewed as a brightness enhancer for diode lasers. Although diode lasers have electrical efficiencies of 70 percent or higher, they generate low-brightness, divergent beams. Even so, diodes serve as efficient pumps for fiber lasers, which effectively convert their output into high-brightness, narrow-output beams.
When doped with various active elements, the fiber component can produce a variety of wavelengths in the infrared. Erbium, ytterbium and thulium, for example, generate outputs at 1, 1.5, and 2 μms, respectively.
The flexibility of fiber lasers is particularly exemplified when configured in a master oscillator power amplifier (MOPA) architecture. Such configurations begin with a seed source such as a simple, narrow-linewidth semiconductor laser that can modulate its repetition rate and pulse duration electronically. Injecting the seed pulses from this source into a well-designed MOPA amplifies them to higher power levels without compromising any of the base qualities of those pulses.
This is not as trivial a design task as it sounds. MOPAs can generate sufficient power to self-lase, to distort the pulse and to degrade spectrally. So care must be taken to avoid such issues as well as the spontaneous emission in the amplifier chains. In a well-designed system, however, the pulse duration, peak power, spectral content, polarization and beam quality are all at your fingertips