From single-molecule studies to in vivo imaging, FRET spectroscopy is a versatile and far-reaching tool. But further work needs to be done before the technique becomes more universally applicable.
Although FRET (Förster resonance energy transfer) is now a mature and established technique, it can always be faster, brighter, more sensitive, more multiplexed and more selective. So research never ceases to extend FRET’s capability and to expand the range of applications for which it can be used.
Improvements to the technique would not only make it easier to use in existing applications, but also open it up to novel applications such as single-molecule analysis inside living cells. Visualizing interactions, conformations and dynamics in their natural biological contexts is still in its infancy, but new developments in internalization procedures, in vivo labeling and fluorescent probes will make this a reality.
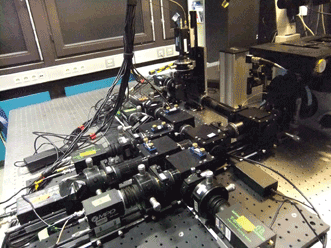
Highly sensitive photon-counting detectors are the centerpiece of most multiparameter FRET spectroscopy techniques. They permit detecting the arrival of single photons with picosecond time resolution. Shown is a custom-built setup featuring eight photon-counting detectors that permit simultaneous detection of various colors and polarization directions. Courtesy of the Lemke Group.
Another game changer will be to combine FRET with other single-molecule techniques such as magnetic tweezers, optical tweezers and atomic force microscopy. “Such combinations will allow combined global (through a force-based method) and local (through FRET) views of a system, as well as provide the ability for nanomanipulation of a molecule while monitoring changes in its structure and conformation,” said Dr. Achillefs Kapanidis, professor of biological physics and head of the Gene Machines Group at Oxford University in England.
FRET probes
The design of FRET probes is still considered an art rather than a science. Crucial to the efficacy of FRET is the selection of the right probes – and developing new ones tailored to the biological system under scrutiny.
“Already using all the FRET tools that exist offers incredible possibilities,” said professor Dr. Niko Hildebrandt of the Nano BioPhotonics Group at the Institute of Fundamental Electronics at the University of Paris-Sud in Orsay, France. “The challenge is always to find the good FRET pair (or pairs) for the desired application and to make it work (or to find that it does not). So the main challenge is to use existing FRET pairs and to integrate them into applications which can benefit from the versatility of FRET.”
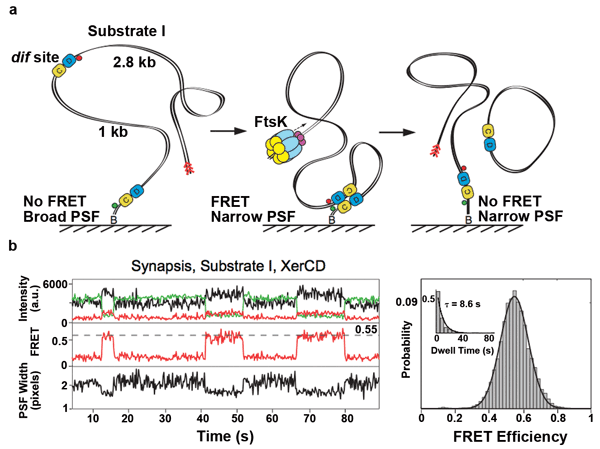
Combining FRET with tethered fluorophore motion offers insight into chromosome segregation. (a) Schematic of the recombination reaction with a DNA substrate for recombination. The DNA substrate has the fluorophores attached to DNA adjacent to each recombination site (dif site); the positions of the acceptor and the donor fluorophores are indicated with red and green circles, respectively. Progression through the recombination was monitored using two observables: Image width (referred to as PSF width) of the acceptor and FRET. Recombination between two dif sites leads to a formation of product DNA molecules; one of them remains attached to the slide and has fluorophores flanking dif on both sides. (b) Nonproductive synaptic complexes (top). Representative time trace of the intensities of donor (green) and acceptor (red) under donor excitation; and acceptor-under-acceptor excitation (black). FRET efficiency (middle) and PSF width (bottom) are shown. The nonproductive events are defined by the ultimate broadening of the PSF coincident with the disappearance of the FRET signal. All data were
acquired at a frame rate of 10 Hz, unless otherwise stated. Histogram of FRET efficiency (right) and
dwell time (inset) of XerCD-dif synaptic complexes (n = 380). Dwell times were fit to single exponentials.
Courtesy of Pawel Zawadzki and Peter May.
When it comes to fluorescent proteins, we are approaching an optimum in terms of spectroscopic properties, according to Raik Grünberg of the Institute for Research in Immunology and Cancer at the University of Montreal. “Several recent variants (such as mTurquoise2) have more than 90 percent quantum efficiency [and] mono-exponential decay behavior, and are fairly photostable,” he said.
In recent years – due to the work of groups led by, for example, Markus Sauer and Thorsten Seidel of Bielefeld University in North Rhine-Westphalia, Germany; Philip Tinnefeld of the University of Brunswick Institute of Technology in Lower Saxony, Germany; and Xiaowei Zhuang of Harvard University in Cambridge, Mass. – we have gained a better understanding of how popular additives modulate the bleaching and blinking behavior of organic fluorophores. Along those lines, there has also been some speculation about self-healing dyes, where an additive is covalently coupled to the dye, as recently introduced by the lab of Scott Blanchard at Weill Medical College of Cornell University in Ithaca, N.Y.
Further understanding the blinking and bleaching mechanisms and how to control them will advance the field, especially when these processes can be realized inside a living cell or organism.
Another option that is gathering interest is the use of gold, which doesn’t bleach or blink. “The combination of DNA nanostructures with the use of gold nanoparticles for fluorescence enhancement of organic dyes [from Tinnefeld’s laboratory] is really exciting, especially when practical and facile ways for coupling labeled biomolecules to the DNA-gold nanostructures are available,” Kapanidis said.
Although fluorescent proteins continue to be the workhorse for FRET measurements in cells, it seems we should see an increase in the use of organic-dye FRET constructs (proteins or nucleic acids) delivered in either bacteria or eukaryotic cells with various means of internalization. These constructs will go beyond stating whether two proteins interact – as in the case of fluorescent-protein-based FRET – and will allow monitoring of structure, interactions and dynamics of molecules that exhibit different diffusion properties in living cells.

One of the main challenges is site-specific protein labeling, a complicated process, especially for large proteins. Before FRET can even be applied, much work needs to be done to the sample to prepare it, which can be both time-consuming and labor-intensive, but the result is very specific, as only the protein of interest is labeled.
When it comes to labels, size matters to Dr. Edward Lemke, who heads the Lemke Group in the Structural and Computational Biology unit at the European Molecular Biology Laboratory in Heidelberg, Germany. And the smaller the better, as far as Lemke is concerned. Currently, FRET probes distances of 3 to 10 nm, and to use FRET as a molecular tool, the labels have to shrink in size.
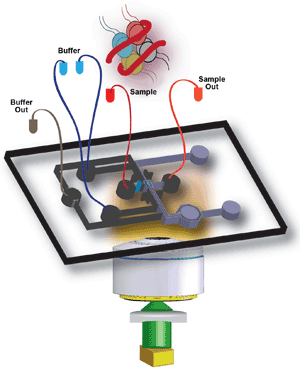
This illustration shows a SWIFT device to which, for example, nucleosomes (also shown) can be
loaded via the sample inlet. Courtesy of the Lemke Group.
Strategies that reliably allow the site-specific introduction of small synthetic fluorophores into proteins in live cells would mark a major advance, and systems for the genetic incorporation of unnatural amino acids may hold big promise.
By streamlining unnatural amino acid mutagenesis (for example, in combination with click chemistry, in which substances are generated quickly and reliably by joining small units together), labeling will become more predictable and efficient.
“Such advances will allow generation of sets of labeled proteins that will permit generation of several distance restraints for getting FRET-based solution structures for large and flexible proteins and their complexes, something that is quite challenging to do using standard structural biology techniques such as x-ray crystallography and NMR [nuclear magnetic resonance],” Kapanidis said.
Also on the horizon for its potential to take FRET to the next level is multiplexing – this refers to simultaneous FRET with different FRET pairs. Hildebrandt and colleagues Igor Medintz at the US Naval Research Laboratory in Washington and Russ Algar at the University of British Columbia in Vancouver, Canada, are exploring the detection of different biomarkers and the simultaneous measurement of different interactions (e.g., protein-protein interaction in and on cells), which are most often functions of diseases.
“Such multiplexing can be made spectrally (distinction of different colors), but also temporally (looking at the photoluminescence lifetimes),” Hildebrandt said.
Measuring two FRET pairs (or more) simultaneously is useful for looking at signaling cascades, but to be able to measure FRET dynamically – i.e., to make FRET movies – would also take FRET to the next level, according to Dr. Klaus Suhling in the physics department at King’s College London.
“FRET movies, not only of one donor-acceptor FRET pair, but of two, so you could tell whether one protein interaction event comes before or after another one – so to see whether one interaction triggers another,” he said. “The imaging technology to make FRET movies has to be perfected. FLIM [fluorescence-lifetime imaging microscopy] movies of FRET are, at present, tricky to obtain with good sensitivity.”
For work where the size of the probe is less important, such as tracking studies on cellular membranes and in vitro motility assays, large fluorescent donors such as quantum dots and upconversion nanoparticles will be an attractive option for biosensing.
“The largest distance over which FRET can be measured is with quantum dots and lanthanides, with a Förster radius of over 10 nm,” Suhling said. “The brighter the donor, the better – but as the quantum yield of many donors is high already, improvements in brightness will be limited, as the quantum yield can only ever be a maximum of 100 percent. More photostable fluorophores will, however, be very useful for prolonged studying of protein interactions or conformational changes.”
FRET challenges
One of the biggest headaches for FRET users is the sample preparation, which requires routine molecular biology/biochemistry and cell biological work that is both slow and labor-intensive. But lab-on-a-chip technologies could alleviate some of the drudgery.
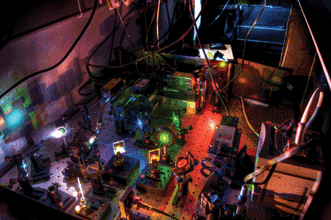
The excitation path of a home-built microscope capable of three-color single-molecule FRET and alternating-laser excitation (ALEX) microscopy. The microscope uses several visible lasers (405, 473, 532, 561 and 640 nm); the modulation and intensity of the lasers are controlled using an acousto-optical tunable filter. The same microscope can be used for localization-based superresolution imaging techniques. Courtesy of Justin Pinkney.
Lemke and colleagues recently published a possible way to overcome this in their latest paper, which appeared in Nature Methods (doi:10.1038/nmeth.2809) in January. Their automated microfluidic platform performs multisecond observation of single molecules with millisecond time resolution while bypassing the need for immobilization procedures. The biomolecules are confined to a thin excitation field by reversibly collapsing microchannels to nanochannels.
Currently, many single-molecule FRET measurements still rely on home-built instruments that are complex and expensive, and require in-house written software, which has limited the wide availability of single-molecule FRET.
“The interest in single-molecule fluorescence in general – driven by the immense interest of biologists in localization-based superresolution imaging – will provide the large market needed to push innovation to the level needed to provide the required instruments that will further increase the popularity of single-molecule FRET, and FRET in general,” Kapanidis said.