Förster resonance energy transfer microscopy is not bound by the same restrictions as conventional light microscopy – it goes beyond the diffraction limit, allowing molecular interactions to be visualized with greater resolution than ever.
The resolution of conventional light microscopes is limited by the wavelength of the light used to illuminate the specimen. FRET (Förster resonance energy transfer) microscopy, on the other hand, detects the consequence of the direct transfer of excitation energy from one fluorophore to another nearby molecule. This enables us to see interactions between molecules or conformational changes within molecules at distances of less than 10 nm, far below the typical diffraction limit of light microscopes at around 200 nm.
First demonstrated more than 50 years ago, FRET is an interaction of two fluorochromes, whereby one transfers the excitation energy directly to the second without the emission of a photon.
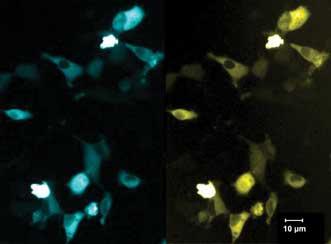
HT-1080 cells seeded and transiently transfected via lipofection with eCFP-epac1(human)-eYFP (pcDNA3-vector) four hours after seeding. FRET imaging was initiated 18 hours after transfection. Courtesy of Eilert et al, Bio Imaging Center Munich.
“FRET is a process through which energy absorbed by one fluorophore (the donor) is transferred directly to another nearby molecule (the acceptor) via a nonradiative pathway,” said professor Richard Day of the Department of Cellular and Integrative Physiology at Indiana University School of Medicine. “This transfer of energy depletes the donor’s excited-state energy, quenching its fluorescence emission, while causing increased emission from a fluorescent acceptor (called sensitized emission).”
The efficiency of resonance energy transfer depends on how well the emission spectra (photon energy) of the donor overlaps the excitation spectra (photon energy) of the acceptor, and how well their electromagnetic dipoles are aligned.
The remarkable resolving power of FRET microscopy opens us up to another world of biological processes. Since the distance over which efficient energy transfer can occur is limited to less than 80 Å, FRET microscopy is one optical method that can achieve the angstrom-scale resolution necessary to monitor the interactions of proteins inside living cells.
“Protein-protein and protein-DNA interactions, as well as protein conformational changes or RNA conformation changes, can be measured with high precision in response to cellular events,” said James Joubert, an applications scientist at Photometrics of Tucson, Ariz. Photometrics manufactures EMCCD and CCD cameras for life sciences research.
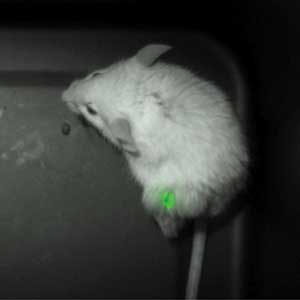
Takeharu Nagai and colleagues used Photometrics’ Evolve 512 electron-multiplying CCD camera to visualize NanoLantern signals in a mouse. Courtesy of The Institute of Scientific and Industrial Research at Osaka University.
Changes in FRET signals can also report subtle conformational changes in probes that are designed to monitor intracellular activities. For example, highly sensitive and specific biosensors for calcium, action potentials, cAMP, ribose, oxygen and other molecules of interest can be constructed from a FRET donor-acceptor pair connected by a binding domain and used to monitor events within living cells.
“A benefit of FRET imaging is that the distances measured are based on a molecular frame of reference, rather than an instrumental one, which is more prone to instrumental noise, drift and aberrations,” Joubert said. “Thus, as FRET is an established technique for monitoring molecular interactions at the nanometer scale, it is often used to confirm colocalization results generated from other nanometer-scale techniques, such as two-color superresolution.”
Today’s FRET instruments are continually improving. For example, at microscopy and imaging system specialist Till Photonics GmbH, an FEI company in Gräfelfing, Germany, the most widely adopted improvement in recent years is that of image splitters that allow simultaneous imaging of the donor and acceptor emission on a single camera chip. Compared with sequentially changing emission filters, using these image splitters is fast, efficient and reliable.
“These image splitters are accompanied with software that can treat the split images as two channels at the point
of acquisition so as not to be cumbersome for processing,” said Jeff Reece, senior technical sales engineer at
Till Photonics. “An example is the Till iMIC microscope with Dichrotome, supported by Live Acquisition software.”
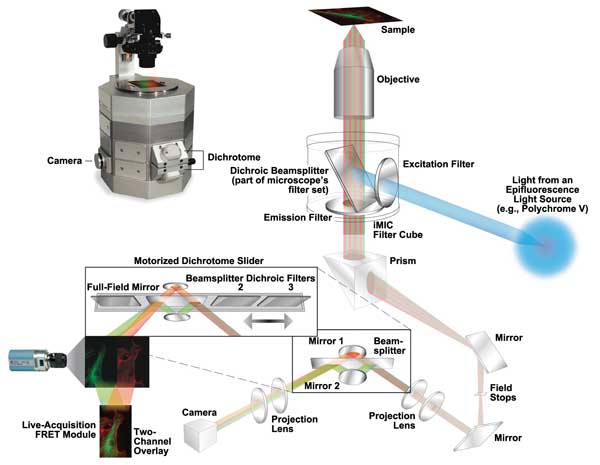
The iMIC microscope and the Dichrotome Dual Emission Extension create a FRET microscope that can track multiple FRET pairs. Courtesy of Till Photonics.
An important boost to the potential of FRET microscopy came about with the widespread application of TIRF (total internal reflection fluorescence) microscopy and the advent of back-thinned electron-multiplying CCD cameras. The result was a major advance in the field, leading to the development of single-molecule FRET (smFRET) for studying populations of individual molecules.
The increased availability of powerful single-wavelength lasers for efficient donor excitation has also improved FRET imaging significantly by reducing the amount of cross-excitation of the acceptor and increasing the photon flux emitted, needed for faster measurements to record shorter-lifetime biological events.
But perhaps the most significant advance that has invigorated the field is the discovery of fluorescent proteins (FPs). Scientists continue to work tirelessly to expand the repertoire of fluorescent proteins, which has led to a dramatic increase in the use of FRET microscopy.
Through the use of mutations of fluorescent proteins and careful testing of the resulting fluorescent protein mutants, pairs with greater FRET efficiency have been developed.
Roger Tsien (now a professor in the Department of Chemistry and Biochemistry at the University of California, San Diego), who is renowned for his groundbreaking work on green fluorescent proteins, and Michael Davidson at Florida State University in Tallahassee recently reported in a Nature Methods paper on improved FRET donor-acceptor pairs.
Their pairing of the brightest green and the brightest red fluorescent proteins showed remarkable photostability and dynamic range, compared with the most commonly used FRET pairs: cyan and yellow.
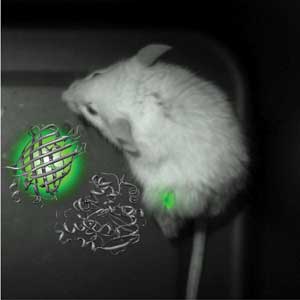
NanoLantern is a bright luminescent protein that allows real-time imaging of intracellular structures in living cells. Courtesy of The Institute of Scientific and Industrial Research at Osaka University.
The paper demonstrated the use of the new FRET pair to detect single action potentials as well as activation of the RhoA GTPases with high efficiency.
The future of FRET
Future applications will need new FRET probes with improved brightness and dynamic range. These probes will come from sources ranging from fluorescent proteins and organic dyes to quantum dots, gold nanoparticles and upconversion nanoparticles.
“Quantum dots have high resistance to photobleaching, large emission intensity, broad excitation spectra and narrow emission spectra, which are ideal for FRET probes,” said Photometrics’ Joubert. “Their broad excitation spectra allow a single wavelength to excite multiple possible donors, and narrow emission bands reduce crosstalk with acceptors.”
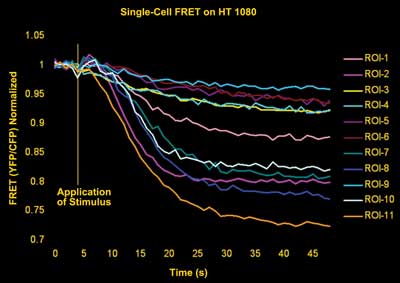
Normalized FRET kinetics of eCFP-epac-eYFP transfected HT-1080 cells.
Cells were stimulated with an adenosine receptor agonist four seconds
after the experiment was initiated. Activation of adenosine receptors
led to an increase in cAMP level in the cytoplasm that can be observed
as a decrease of FRET signal. Courtesy of Eilert et al, Bio Imaging
Center Munich.
There is also considerable interest in developing near-infrared-emitting FPs for studies inside living animals.
“The development of new FRET pairs that are excited and emit further into the red and near-IR will also help reduce phototoxicity, background fluorescence and scatter from thicker samples,” Joubert said. “An increasing multitude of combinations of biomolecules-binding partners – including protein-protein, protein-DNA, protein-RNA, protein-lipid and so on – will be studied via FRET. Newer FRET biosensors will be developed to detect an even larger array of key biological signaling molecules.”
Techniques that require measuring several wavelengths at the same time, and fast, are becoming more and more prominent, according to Maria Marosvölgyi, the primary applications specialist at Till Photonics.
“The combination of several FRET pairs for triple FRET or multiple FRET is facilitated by automatic and fast switching of FRET filter sets as in the Till-FEI Dichrotome iMIC system,” she said.
Adam Hoppe, assistant professor at South Dakota State University in Brookings, is working on extending FRET to imaging multiple protein interactions in living cells using a method called N-way FRET analysis. Hoppe believes that the demonstration of more than three colors (and their combinations) engaged in FRET is just on the horizon.
“If up to four colors can be monitored, as many as six protein-protein interactions can be measured with high spatiotemporal resolution,” he said. “This idea, if applied to questions about signal transduction, would give an unprecedented view of signal cluster dynamics and would continue to push the frontiers of fluorescence microscopy forward.”
With the implementation of mathematical image processing methods, the capabilities of and resolution of FRET microscopy are expanding.
Another avenue of research for Hoppe includes 3-D reconstruction of FRET microscopy data, which, along with
N-way FRET, promises to enable imaging of three or more protein interactions throughout the 3-D space of living cells
at unprecedented resolution.1
References
1. A.D. Hoppe et al (July 2008). Three-dimensional FRET reconstruction microscopy for analysis of dynamic molecular interactions in live cells. Biophys J, Vol. 95, pp. 400-418.
2. J. Zhang et al (2002). Creating new ?uorescent probes for cell biology. Nat Rev Mol Cell Biol, Vol. 3, pp. 906-918.
3. L.M. DiPilato and J. Zhang (February 2010). Fluorescent protein-based biosensors: resolving spatiotemporal dynamics of signaling. Curr Opin Chem Biol, Vol. 14, pp. 37-42.
4. A. Miyawaki (October 2011). Proteins on the move: insights gained from fluorescent protein technologies. Nat Rev Mol Cell Biol, Vol. 12, pp. 656-668.
5. Y. Lu et al (2003). New highly sensitive and selective catalytic DNA biosensors for metal ions. Biosens Bioelectron, Vol. 18, pp. 529-540.
6. J. Ben Arous et al (March 2010). Automated imaging of neuronal activity in freely behaving Caenorhabditis elegans. J Neurosci Methods, pp. 229-234.
7. E. Kardash et al (2011). Imaging protein activity in live embryos using fluorescence resonance energy transfer biosensors. Nat Protoc, Vol. 6, pp. 1835-1846.
8. M. Hara et al (2004). Imaging endoplasmic reticulum calcium with a ?uorescent biosensor in transgenic mice. Am J Physiol Cell Physiol, Vol. 287, pp. C932–C938.
9. Y. Kamioka et al (2012). Live imaging of protein kinase activities in transgenic mice expressing FRET biosensors. Cell Struct Funct, Vol. 37, pp. 65-73.
The biosensor probe
Professor Richard Day of the School of Medicine at Indiana University explains an interesting application of FRET microscopy:
“Originally envisioned by Roger Tsien … biosensor probes are genetically encoded proteins with modular design that includes a sensing unit that is directly linked to reporter units. Generally, the reporter unit consists of a pair of FPs that share the significant spectral overlap required for efficient FRET.
“The sensing unit serves as a linker between the FPs, and includes an element that is modified by the targeted biological event, and a binding motif that recognizes the modification. This allows the sensing unit to change its conformation in response to a specific signaling event, altering the distance between the FP pair. The changing intramolecular FRET signal from these single-chain biosensor proteins can be monitored in real time, allowing measurement of the spatiotemporal dynamics of protein activities2-4…
“The transgenes encoding these probes have been successfully introduced into a variety of organisms. For example, calcium-sensing biosensor proteins have been used to image biological activities in living C. elegans, Drosophila and zebra fish.5-7
“The imaging of biosensor activities in transgenic mice, however, has proved problematic, and this may be related to the low-level expression of the proteins caused by transgene silencing.8 Recently, a transposon-mediated gene transfer method was used to generate transgenic mice with a high level of biosensor expression. This allowed the activity of these reporters to be monitored in several different tissues by two-photon excitation microscopy.”9