Advances in compact EUV lasers could benefit industries from semiconductor manufacturing to medicine.
Compact lasers that operate in the extreme ultraviolet (EUV) wavelengths – 5 to 50 nm – are poised to offer new abilities to image, pattern and manipulate objects, thanks to short wavelengths and energetic photons.
Researchers are working on EUV lasers at Colorado State University in Fort Collins – one of four core partners of the National Science Foundation’s Engineering Research Center for Extreme Ultraviolet Science and Technology – at the University of Bern in Switzerland, and elsewhere. If they are successful, industries from medicine to semiconductor manufacturing will benefit. The prospect has observers excited.
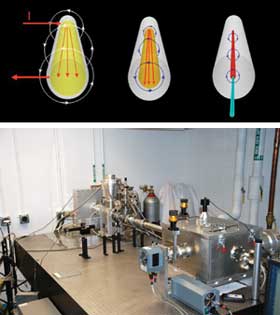
(Top) In a capillary-discharge extreme ultraviolet (EUV) laser, the magnetic field from a fast current pulse (indicated by I in the first stage to the left) rapidly compresses the plasma toward the axis, creating hot and dense columns with aspect ratio length/diameter >1000. Lasing occurs close to the maximum compression. (Bottom) An EUV laser (background left) mounted on a microscopy test bed (right). Courtesy of Fernando Tomasel, XUV Lasers Inc.
“The new frontiers are the photon sources in the soft x-ray and the EUV areas and the applications coming out,” said Vivek Bakshi, president of the Austin, Texas-based consultancy EUV Litho Inc.
Bakshi refers to sources, not lasers. In part, that’s because today’s lasers produce, at best, a few tens of microwatts of pulse power. The 13.5-nm EUV lithography being pursued by the semiconductor industry (see “Plasma Source Enables High-Volume Manufacturing with EUV Lithography,” Photonics Spectra, September 2011, p. 59) requires hundreds of watts of in-band power. Thus, available EUV lasers will not power lithography tools for many years to come – if ever.
However, EUV lasers could be useful elsewhere in semiconductor manufacturing because they enable imaging with great resolution. Currently, the industry is inspecting masks; for example, using lasers operating at 193 nm, a situation that cannot last because process dimensions continue to shrink.
“There is a point where you have to switch over to EUV because the features get so small,” said Stefan Wurm, director for lithography at the semiconductor industry consortium Sematech.
Mask defect review using a 13.2-nm EUV laser has been demonstrated, although the throughput was too low to be commercially viable. To fix that, more photons are needed.
That also is being worked on at a longer wavelength by XUV Lasers Inc. of Fort Collins, which manufactures desktop-size capillary discharge lasers that output light at 46.9 nm. High average power often is requested by the company’s customers, said Fernando Tomasel, director of laser systems. XUV Lasers currently is developing a system that will run faster than 10 Hz.
The short wavelength of the source offers advantages, Tomasel said. “You can focus the laser into a small spot, on the order of 50 nm.”
This makes it possible to ablate a sample, such as a microorganism, and study its chemical composition at different spots, and renders its data of value to biology and medicine. Other EUV laser research applications include patterning, imaging and photochemistry.
The first is being pursued by Mario Marconi, an electrical and computer engineering professor at Colorado State. He has implemented an extreme ultraviolet version of an 1836 discovery. The technique uses a mask composed of regular, repeating elements arranged in a matrix. Coherent light traveling through the mask replicates the pattern in a plane where photoresist can capture it. The method offers a key advantage over alternatives.
“If you have a defect in one element of the mask, the relationship between the area of this defective element to the total mask is so small that the defect will not show up in the print,” Marconi said.
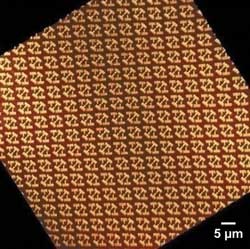
Talbot printing done with an EUV laser allows nanoscale patterning of repetitive arrays that is largely immune to mask defects. Courtesy of Mario Marconi, Colorado State University.
In a recent experiment, researchers exposed a mask with an entire row of defects. The substitution had no effect. The scientists have printed 130-nm features over areas that measure hundreds of microns across. A paper on this appeared in the November/December 2009 Journal of Vacuum Science and Technology, and another is pending. The technique has been demonstrated with 46.9-nm lasers, and Marconi believes that it will be relatively easy to translate it to a shorter wavelength when higher intensity sources become available.
Carmen Menoni, another Colorado State professor, is using EUV lasers to build full-field imaging microscopes with a spatial resolution down to 30 nm. She has demonstrated single-shot imaging with a 46.9-nm source, tracking events on the nanoscale with what is essentially a strobe light.
“You have these flashes that freeze the motion at a given instant in time. The duration of the pulse is what determines the window in which you take the image,” she said.
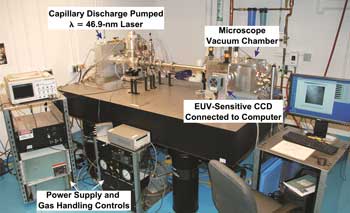
This 46.9-nm laser allows single-shot full-field microscope imaging of nanostructures ranging from biological samples to semiconductors. Courtesy of Carmen Menoni, Colorado State University.
The technique can record repetitive phenomena in cantilevers, membranes and other microelectromechanical systems constructs. These are used in a variety of ways and devices. Being able to image them could benefit industry as well as researchers.
To work in single-shot mode, the microscope needs an adequate photon flux, such as that provided by an EUV laser. As with other EUV applications, the microscope operates in a vacuum because extreme ultraviolet is absorbed by nearly everything. For the same reason, EUV optics often use reflectors, typically constructed out of multilayer materials. The interference between layers gives rise to reflectivity of about 70 percent, at best.
For imaging, one area of attention is on photons in the 3-nm range, where there is a water window created by differential absorption of oxygen and carbon. This allows a natural contrast between a biological specimen and the water that surrounds it.
Davide Bleiner, an assistant professor in applied physics at the University of Bern, heads up a group looking at such applications. He is working with fellow Bern professor and longtime EUV researcher Jürg Balmer, who developed the compact source used by the group.
These devices fire laser pulses onto a solid target. The initial ones ablate the target and create a plasma, and they are followed by a main driving pulse. This arrives at an angle, producing a wave that travels through the plasma at a speed calibrated to improve EUV production efficiency.
Currently, the shortest wavelength possible with such an approach is 8.8 nm, obtained using lanthanum. Cutting the wavelength will require going up in the periodic table and increasing the driver pulse energy. That could prove prohibitive in a laboratory-scale installation for high-atomic-number elements. Some standard techniques to cut wavelength can’t be used because of their impact on imaging and spectroscopy applications.
“We don’t want to do harmonics because that would provide poor EUV photon fluxes and broaden the spectrum, such that we would lose probing selectivity and contrast,” Bleiner said.
Elliot R. Bernstein, a professor of chemistry at Colorado State, uses EUV lasers because the photons pack a punch and ionize virtually any material. This allows researchers to unravel chemical reactions by analyzing the initial compound, the intermediate products and the final result.
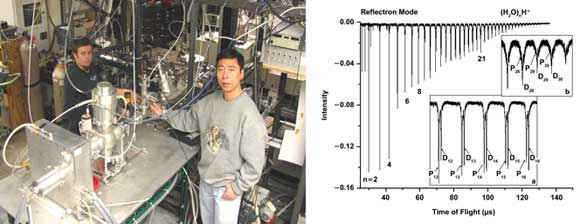
An EUV laser (left) ionizes water clusters, yielding their mass spectrum (right) and composition. Courtesy of Elliot Bernstein, Colorado State University.
Bernstein and his group are interested in the decomposition of energetic materials, a substance class that includes rocket fuel and explosives. Although rockets fly and bombs burst, intelligently designing a new version of what causes either can be challenging because the precise details of how energy is produced may be obscure.
“If you want the energy to be released more slowly or more rapidly or in a different format, it’s very important to know what’s actually happening, how the decomposition takes place,” Bernstein said.
He added that, although the current crop of 46.9-nm EUV lasers work well for his application, he would like to have systems that fire faster to speed up experiments.
As for the future, EUV lasers are not the only sources below 50 nm. Systems based on high-order harmonics are being developed at the University of Colorado at Boulder, another core partner of the National Science Foundation’s EUV center, at Switzerland’s National Center of Competence in Research Molecular Ultrafast Science and Technology, and elsewhere. For its part, the semiconductor industry is investing heavily in incoherent plasma sources. Compared with EUV lasers, the first offers a shorter pulse width, wavelength tunability and higher repetition rates. The second can provide magnitudes more power.
However, both alternatives also suffer from disadvantages when compared with EUV lasers. High-harmonic sources produce lower energy and spectrally broader pulses. Plasma sources are limited in their peak brightness.
What’s more, EUV lasers have been making steady advances. Thus, they could be ready to move out of the lab and into applications. Gain-saturated systems that produce enough photons to be useful have been demonstrated from 46.9 down to 8.8 nm.
There also is an increasing emphasis on making systems smaller, notes Jorge Rocca, a professor at Colorado State who also is director of the NSF’s Engineering Research Center for Extreme Ultraviolet Science and Technology.
“There has been a lot of progress in the field, expanding the wavelength range and also making these lasers more compact,” Rocca said.
Measuring Your EUV Laser
Erik Schoeffel, McPherson Inc., [email protected]
For manufacturers and others who have acquired extreme-ultraviolet (EUV) lasers recently, some test and measurement are in order. At the very least, you will want to measure the exact wavelengths emitted. Perhaps you will want to select one wavelength and use it to interact with a sample in some experiment. Both jobs call for spectrometers.
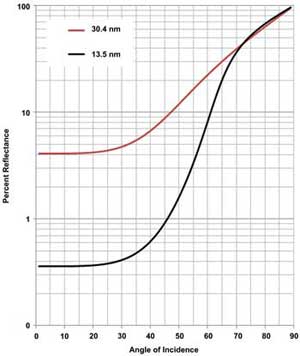
Figure 1. Reflection of gold vs. angle of incidence.
At short wavelengths, designs such as the Czerny-Turner are not viable. This spectrometer has at least three reflective surfaces, all at near-normal incidence angles. Even with a vacuum enclosure and state-of-the-art reflective coatings, it is not useful at wavelengths of <110 nm.
Fortunately, designs exist that use diffraction gratings on concave substrates. These reduce the optical system to one reflective surface that simultaneously disperses and focuses spectra. In a suitable vacuum enclosure, they work to wavelengths of slightly <30 nm, even when using near-normal incidence angles. Spectral diagnosis of laser output wavelengths is relatively fast and easy with these instruments. Appreciable wavelength regions in the vacuum-UV (VUV) can be measured simultaneously when equipped with microchannel plate intensifiers or direct-detection CCD detectors. These designs also are useful for scanning monochromator applications and for “dialing in” a particular wavelength for subsequent focus optics and sample illumination.
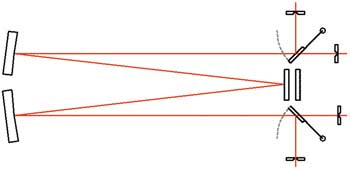
Figure 2. Czerny-Turner design uses multiple reflective surfaces and vacuum enclosure for wavelengths of >110 nm.
Spectrometers for work at wavelengths of <30 nm also may use diffraction gratings on concave substrates. Angle-of-incidence changes from normal to grazing boost reflective efficiency (see Figure 1, the chart of modeled data, which underscores the requirement for use of spectrometers in the soft x-ray and EUV, particularly when multiple optics are used). By using a 2° or 3° grazing angle of incidence, reflective efficiency can be >80 percent at wavelengths as short as 8 nm.
Some soft-x-ray and EUV spectrograph gratings have been designed and produced for dedicated regions. They can render spectral measurements of laser output in a fixed region relatively simple. More versatile, Rowland circle grazing incidence spectrometers are analytical tools for greater wavelength regions and can work as scanning monochromators or spectrographs, depending upon selected detection equipment; however, neither is ideal for dialing in an EUV wavelength and illuminating a sample.
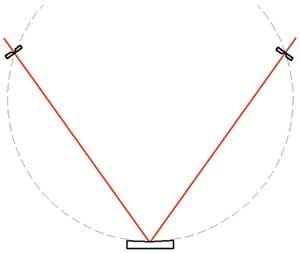
Figure 3. Seya-Namioka design uses concave diffraction grating to simultaneously disperse and focus. Useful for wavelengths of >30 nm.
To accomplish this, more complex instruments with concave spherical, toroidal or off-axis parabolic collimation and focusing mirrors, in combination with plane diffraction gratings, often are used. These can become quite large, complex and, eventually, “beam line” instruments. Because optical aberrations contribute significantly to instrument performance at grazing incidence angles, care must be taken to adopt suitable designs and to plan for the best possible optics. Done well, these select a discrete EUV laser wavelength with good spectral resolution and focus to a sample; e.g., in a photoelectron spectrometer.
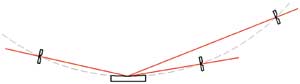
Figure 4. Rowland circle grazing incidence design works at 2° or 3° angle of incidence and is useful for wavelengths of >1 nm.
Another important design consideration occurs when pulse broadening is of concern. A diffraction grating, rotated to tune for a particular wavelength, can introduce appreciable broadening of narrow laser pulses. To minimize the broadening introduced by rotation of a single diffraction grating, spectrometers may be built as doubles. Two instruments work together to select wavelengths, while their mirrored optical paths negate broadening effects. In recent years, developments include double off-plane grazing incidence instruments that further minimize residual broadening effects.
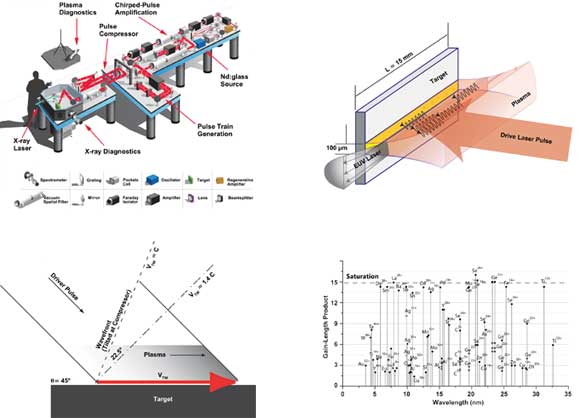
In the Bern Advanced Glass Laser for Experiments at the University of Bern in Switzerland (a), a drive laser pulse (b) applied at the optimum angle (c) produces EUV photons most efficiently and at wavelengths down to 8.8 nm. The wavelength is set by the material from which the target is made (d), limiting tunability – a limitation in all EUV laser schemes. Courtesy of Davide Bleiner, University of Bern.
Spectrometers currently are available for much of the soft x-ray, extreme UV and VUV regions, although not always in the form in which we are accustomed to seeing. Selecting a spectrometer requires prioritizing analytical goals, wavelength range, spectral resolution, dispersion and possibly optical aperture requirements in light of experimental needs. Shorter wavelengths require ever more care in selection of optical schemes to maintain efficiency and performance.
The optical designs pictured in Figures 2 through 4 demonstrate the progression from the multisurface normal-incident Czerny-Turner – often used for visible spectroscopy – to the single-surface grazing-angle spectrometers – useful in the soft x-ray and EUV regions.