LED arrays, specialized lasers, and a gamut of sensors mark the newest biocompatible wearable devices, capable of detecting the early onset of cancer and other diseases.
The next generation of wearable devices will no longer be created only for the fitness-conscious to monitor their daily steps or heart rate on a smartwatch. Increasingly sophisticated photonic components such as LED arrays and specialized lasers, as well as a gamut of sensors and detectors contained within mobile equipment attached to the body, will be able to monitor spectral data within both the visible and infrared ranges.
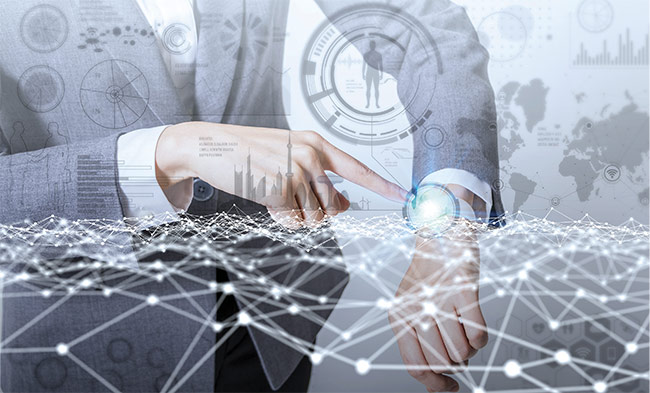
New wearable devices could soon expand remote diagnostics. Courtesy of iStock.com/metamoworks.
These devices, a number of which are approaching clinical trial, have shown the capability to provide valuable medical information, including measuring the distribution of oxygen in blood and tissue while detecting a variety of biomarkers that warn of the arrival of diseases and conditions well before symptoms force a patient to visit a hospital or clinic. Technologies currently being tested include diffuse optics and near-infrared spectroscopy, for example.
Meanwhile, the rapid spread of COVID-19 — and the prospect of future pandemics — has only made this trend toward the mobile collection of health data more urgent. Continuous physiological monitoring of health indicators among all ages and lifestyles may soon provide for testing and therapeutic guidance within or nearby a patient’s own home when office visits are not possible due to travel or visitation restrictions.
ResearchandMarkets reported that the global wearable technology market is expected to reach more than $118 billion and grow at a compound annual rate of 13.8% between 2021 and 2028. The wearable sensors market is divided into categories such as accelerometer, gyroscope, optical, and force and pressure systems. The wristwear design has dominated wearable sales and is projected to do so for at least the next several years.
Athletes in the lead
Sports associations such as the National Collegiate Athletic Association (NCAA) have a long-standing interest in preventing expensive injuries. So they have provided resources to develop portable systems that can measure and establish the optimal use of athletes’ bodies. These efforts have also offered inroads into a variety of medical and general health-related applications, such as analyzing healthy and diseased tissue following diagnosis and subsequent treatment.
A number of trends are driving these changes, including the growing elderly population in the U.S. According to the U.S. Census Bureau, adults age 65 and older will number 77 million by 2034. This demographic will have a variety of needs, including fall risk assessment and prevention, physical activity monitoring, and weight control and disease management. The pandemic has shown that the need to evaluate these types of data remotely only increases when many seniors are confined to their homes.
Systems for continuous evaluation used to be composed of a complex conglomeration of parts, such as wiring and circuit boards, and such systems were bulky and inflexible. These elements complicated the reading of health data by allowing noise and other environmental factors to interfere with data collection. But the miniaturization of label-free, noninvasive diagnostic technology, along with any inherent optical components, has enabled the ability to design increasingly sophisticated wireless and biocompatible wearable devices. Among other upgrades, modern optical wearables capitalize on such innovations as surface-mount light-emitting diodes (LEDs) with many wavelengths in a module, flexible ultraviolet sensors, and skin patches using near-field communication technology1.
In an examination of the range of biomarkers now tracked by optical wearable technology, a team of researchers from Australia, Chile, and India said that, according to a literature review, a wide range of modalities were researched as potential drivers for detecting biomarkers, including plasmonic arrays, total internal reflection dark-field microscopy, and surface-enhanced Raman spectroscopy. The range of biomarkers that could provide vital information on blood oxygenation, as well as more advanced data about the early stages of cancer, can be indicated by changes in skin color, the presence of electrolytes and metabolites, or the presence of lipids, among other indicators1.
“To design and fabricate a compact patch to diagnose these biomarkers using optical technology, we have only two sources, LED and laser,” said Shanmuga Sundar Dhanabalan from the Functional Materials and Microsystems Research Group at RMIT University in Australia and one of the authors of the study. Due to the bulkiness of laser technology, he said, the desire to create a small, wearable patch led naturally to the incorporation of LEDs.
It is now possible to recharge a flexible patch wirelessly, thanks to a surface-mount device-based near-field communication transceiver chip that harvests power and transmits it to the entire patch using a linear voltage regulator. Research is currently underway to harvest power from electromagnetic radiation, which is freely available in the environment. The power could be gathered by a frequency-specific antenna and then converted into direct current using rectifier diodes.
But as the devices have advanced, the underlying challenges have remained the same, said Madhu Bhaskaran, co-leader of the research group and another author of the study. “The basic principle behind this measurement is based on the intensity changes in the incident light due to the presence of the biomarkers,” Bhaskaran said. “Each biomarker responds to a different wavelength and their absorption spectra will be different accordingly.”
Noninvasive monitoring of biomarkers generally focuses on optical information obtained via backscattered light from materials within skin, sweat, and blood. Optical transducers, which convert light rays into an electronic signal, can measure changes in the transmission, absorption, reflectance, and interferometric patterns of incident light. Available data can be gathered from skin color changes; levels of lactate, glucose, creatinine, urea, cortisol, and uric acid in sweat; and oxyhemoglobin, deoxyhemoglobin, carboxyhemoglobin, and sulfhemoglobin in the blood.
In the case of oxy- and deoxyhemoglobin, a red LED at 660 nm and an IR LED at 940 nm are used in a standard oximeter, although ongoing experiments have tested other wavelengths as well. Tests have focused on the effective distance between the LED and the photodiode in a wearable setup. Optical absorption has been established between 200 and
1200 nm for most biomarkers — for example, just over 200 nm for cholesterol, just under 300 nm for uric acid, almost 1200 nm for collagen, and between
260 and 270 nm for glucose.
Oxygenation of tissue
Measurements of oxygen levels in the blood — particularly oxy- and deoxyhemoglobin, which show the flow of oxygen to different parts of the body — are commonplace and serve as key indicators of health and activity within the vascular network. But this method is also indirect, since blood oxygenation is not always a reliable measure of the perfusion of oxygen within the tissue itself.
So a team of researchers from the Wellman Center for Photomedicine at Massachusetts General Hospital and Harvard Medical School recently set out to develop a more reliable option. Last year, they reported on the deployment of an oxygen-sensing film along with machine learning to accurately measure tissue oxygenation through a subject’s skin. The researchers tested the functionality of the system — which works like a wristwatch except that it is placed halfway up an arm or limb — on a Yorkshire swine, in which blood flow in the limbs was temporarily restricted by a tourniquet.
The film was attached to the sensor head using a miniaturized system of electronics (Figure 1) that included a flexible printed circuit board within a 3D-printed casing, two high-power ultraviolet (UVA) LEDs, a PIN photodiode, and a thermistor (resistance thermometer). The data was shown to be highly reproducible and was validated using other technologies in the porcine model2.
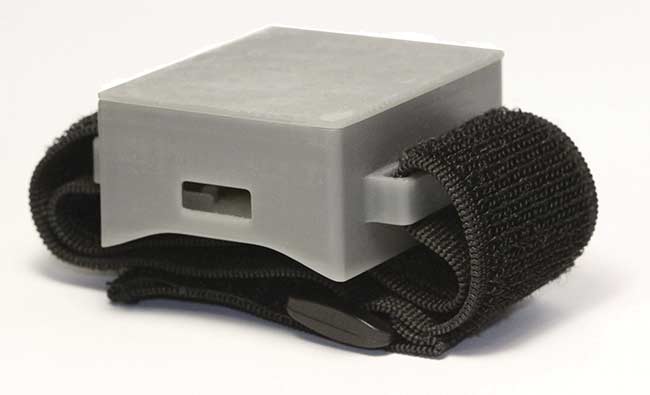
Figure 1. A device, composed of sensors and electronics, that measures tissue oxygenation and that was developed at the Wellman Center for Photomedicine. Courtesy of Conor Evans.
“Our sensors look at pO2, or partial pressure of oxygen, and provide a quantitative value,” said Conor Evans, a professor at the Wellman Center for Photomedicine. An important metric when evaluating oxygenation using the Wellman device is the phosphorescence lifetime, or the average time that the key porphyrin sensor molecule remains excited before photons are emitted, Evans said. “Typically, transcutaneous oxygen measurements are conducted using bulky equipment at the bedside in a clinical setting, requiring trained professionals who can calibrate the equipment. So what we wanted to know was: Can we use optical tools and make our system portable and easy to use?”
Evans’ colleagues have a background in machine learning, which helped to further establish the accuracy of the readings. “When you get the data you need to establish what’s the signal and what’s noise — that’s where machine learning comes in,” he said.
The latest oxygen sensing device is being tested in a pair of human studies, in which enrollment recently wrapped up. Evans said the subjects in the study were directed to complete specific exercises. Then changes in tissue oxygen saturation were measured to monitor changes in oxygen levels in specific regions of the body and on the periphery of the areas, and to monitor how muscles were performing (Figure 2). Much of the work has been funded by the U.S. Department of Defense to ultimately gauge the physical readiness of active duty troops after they have recovered from an injury. Additionally, medical researchers have performed testing following breast reconstruction surgery after cancer treatment, which involved a perforator flap transplantation procedure akin to the reconstruction surgeries faced by many wounded service members.
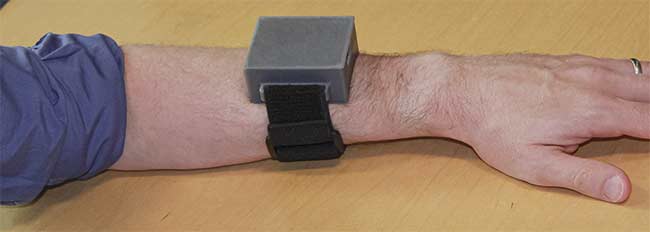
Figure 2. Worn like a wristwatch, the Wellman Center device measures how various muscles are performing in the body. Courtesy of Conor Evans.
“The real benefit will be when devices like this one get to patients,” Evans said. “We are currently matching up with industry partners to commercialize our progress.”
From sport to health support
For the athletic market, measuring the perfusion of oxygen or blood flow into a particular region via a device strapped to a limb can help determine how a specific muscle is doing and whether training or treatment needs to be focused on that area in the body.
“The infrared and near-infrared range are perfect for finding the weak links in oxygenation of blood and muscle and can show an athlete how to improve their technique,” said Kevin McCully, a professor in the Department of Kinesiology at the University of Georgia. “I have been working to adapt a wearable device produced by Train.Red, whose parent company is Artinis Medical Systems, which also uses AI to interpret the data provided.”
The uses of this technology, however, have gradually expanded from athletic training into medicine, focusing on the warning signs for conditions, such as poor circulation or peripheral arterial disease.
McCully’s work has largely centered around the analysis of mitochondria, the membrane-bound organelles that generate energy for normal cell functioning. Muscle fibers with high mitochondrial content are less likely to fatigue. In conjunction with Artinis, he has developed a portable “black box” system on which a clinician — or really anyone — could push a button and receive an accurate measurement of data related to the performance of mitochondria.
In studies completed several years ago, McCully and his colleagues determined that NIR spectroscopy was an effective means of identifying muscular dysfunction in patients with neurological injuries and diseases such spinal cord injury, multiple sclerosis, and amyotrophic lateral sclerosis; cardiovascular and respiratory diseases such as heart failure, cystic fibrosis, and chronic obstructive pulmonary disease; and the mitochondrial disease Friedreich ataxia. Further, researchers found that NIRS data was useful in tracking the effectiveness of various therapeutic interventions because it was capable of identifying differences in oxygen capacity within specific muscle groups3.
“We have envisioned [that] the optical device could be contained in a sock or sleeve,” McCully said.
Projecting spectral wavelengths
One well-known company that has experienced both the successes and the challenges of commercializing wearable technology is Rockley Photonics, which focuses on noninvasive biosensing solutions for the consumer wearables and medtech markets. The company’s silicon photonics integrated circuit chip contains an infrared spectrometer and projects narrow laser wavelengths across a wide spectrum. The system was developed eight years ago, and it is capable of tracking a multitude of biomarkers from a single wearable device (Figure 3).
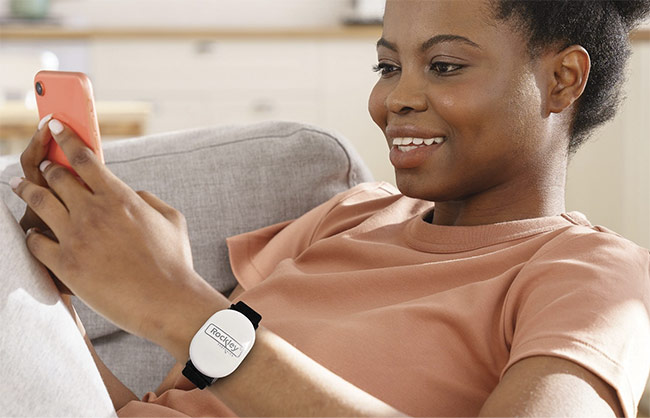
Figure 3. A chip-based infrared spectrometer could measure a wide range of health data via a wristwatch design. Courtesy of Rockley Photonics.
“Our miniaturized technology pro-
jects laser lines across a broad spectral wavelength with remarkable accuracy,” Rockley CEO Andrew Rickman said. “The SNR [signal-to-noise ratio] is actually much stronger than some benchtop systems.”
The Rockley device uses infrared lasers that can track a broader range of biomarkers than can the green LEDs that are commonly used in many health-tracking wearables, and the acquisition time is reduced, Rickman said.
In fact, the company recently partnered with Medtronic to incorporate Rockley’s Bioptx biomarker sensing platform into Medtronic’s device architecture across various health care settings. The goal was to construct a wearable that could measure a number of factors, such as core body temperature, blood pressure, body hydration, and alcohol, lactate, and glucose levels. An AI algorithm would then be deployed to distill the data into an overall health assessment that a clinician and a patient could work from. Late last year, Rockley announced that it had completed a study in which a photonics-based sensor contained within a wristband was used to detect water spectra at various layers of the dermis, giving a more accurate representation of the wearer’s core temperature than common methods that take measurements in the mouth or ear.
According to a recent market report from Iceberg Research, however, the anticipated integration of Rockley’s technology into a popular wrist wearable, particularly for blood pressure and glucose tracking, may be delayed. The company’s stock fell on the news.
The company said that its product development is proceeding on schedule. “We expect to announce additional results from our ongoing human studies for additional biomarkers throughout 2022,” Rickman said. Samples of the platform — complete with wearable band and cloud analytics — have already been shipped to various customers, he said. “Thus far, we have 17 contracted customers, including six of the top 10 consumer wearable companies.”
Implantable sensors
Developers anticipate that in the future these technologies will help to provide early diagnoses of specific diseases or chronic conditions, such as diabetes and cardiovascular disease, and follow the onset and progression of systems in real or near-real time. Continuous monitoring will reveal when a patient’s system has deviated from expected normal functioning.
“Together with colleagues from Texas A&M University, we are working on implantable sensors that are inside the skin (approximately 1 to 2 mm deep), sensing the glucose concentration through the skin using a wearable optical reader,” said professor Aydogan Ozcan from UCLA and the Howard Hughes Medical Institute. “Interstitial fluid is the source of our measurements, and the phosphorescence lifetime is modulated in response to glucose levels, and it [the phosphorescence lifetime] is read through the skin using a wearable reader.”
The research, funded by the National Science Foundation, includes ongoing phantom and animal experiments. The research is moving toward testing on humans in the next phase of the study.
As the understanding of the significance of specific biomarkers increases, the continued construction of miniaturized sensors will attempt to connect success in the laboratory with life in the real world. This will enable patients to live their daily lives while feeling secure that their vital signs are being continuously monitored outside of the doctor’s office.
References
1. S. Dhanabalan et al. (2021). Wearable label-free optical biodetectors: progress and perspectives. Adv Photonics Res, Vol. 2,
p. 2000076.
2. J. Cascales et al. (2020). Wearable device for remote monitoring of transcutaneous tissue oxygenation. Biomed Opt Express, Vol. 11, No. 12, pp. 6989-7002.
3. T. Willingham and K. McCully (2017). In vivo assessment of mitochondrial dysfunction in clinical populations using near-
infrared spectroscopy. Front Physiol,
Vol. 8, No. 689.
Functional NIR Spectroscopy Opens Window into Brain Health
Functional near-infrared spectroscopy (fNIRS) has in recent years been deployed to study brain activity patterns. The underlying principle of the technology is that it tracks hemoglobin inside the brain based on differences in optical absorption. The technique has been deemed both portable as well as usable over the long term by the National Institutes of Health and other organizations.
Researchers at multiple institutions have begun tracking a select group of biomarkers in the brain via a comfortable, wearable headband.
One researcher who is working toward practical product development in the fNIRS arena is Maria Angela Franceschini, a professor of radiology at Harvard Medical School. She also serves as the president of the Society for functional Near-Infrared Spectroscopy.
“When you look at low oxygen perfusion that can be caused by disease, there are cerebral oximeters out there, but they all have a box, cable, and optical probes,” she said. “Our system had to be wireless and made at low cost. And it was important to make something that was comfortable enough for people such as the elderly in a nursing home to wear.”
Called FlexNIRS, the device uses a combination of four LEDs — two dual-color sources at 735 and 850 nm — which work better for head-mounted devices because of their safety with respect to lasers, Franceschini said. In the headband, which is secured by a Velcro strap, three photodiode detectors are set at a range that allows for accurate measurement of factors such as oxygen levels in the blood. The system is powered by a rechargeable battery, and an Android application was created for portable data collection.
In a recently published study, subjects were put through a series of tests that included having them perform activities such as hyperventilating, holding their breath, walking, and climbing stairs. Hemoglobin oxygen saturation was measured accurately at various points around the forehead, and the results were validated by other tests1. Monitoring cerebral oxygen saturation could aid in detecting conditions such as sleep apnea and stroke, as well as cardiovascular diseases.
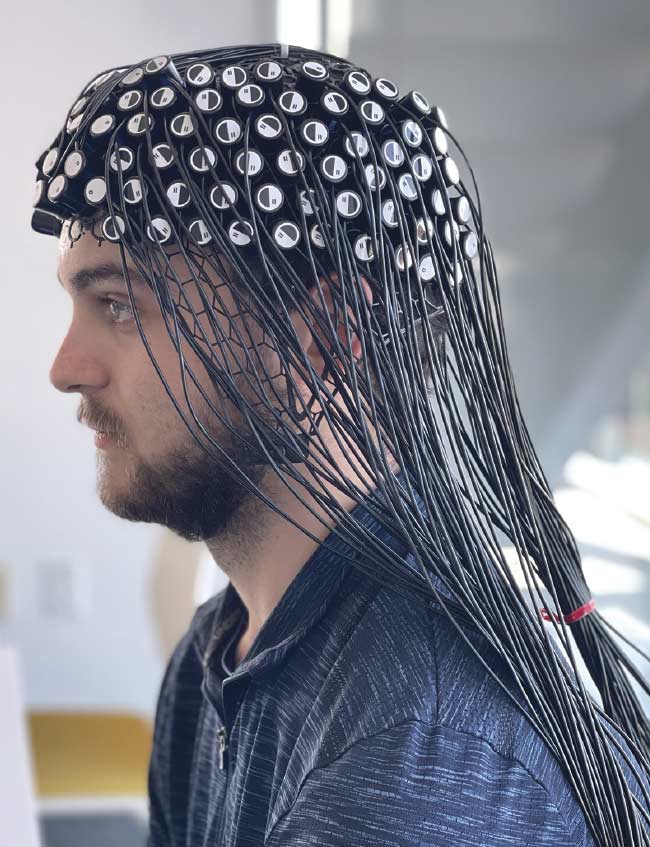
A cap equipped with numerous optodes uses functional near-infrared spectroscopy to measure brain activity. Courtesy of Boston University.
Another company, Kernel, has focused on neurotechnology. It developed a headset for time-domain fNIRS measurements. In this method, picosecond pulses of light are sent into tissue. Time-of-flight measurements of the photons enable tracking of changes in the tissue. Called Kernel Flow, the system contains 52 modules, each with a dual-wavelength laser diode and six detectors — composed of application-specific integrated circuits that measure time of flight — located in four plates strategically placed over the frontal, parietal, temporal, and occipital lobes of the cerebral cortex. A preliminary study showed that the headset was validated by collecting data from human subjects as they performed defined tasks2.
David Boas, director of the Neurophotonics Center at Boston University, expects fNIRS to be a driving force behind the expansion of wearable technology over the next decade. “A brain cognitive interface is a consumer-driven market, which has been of great interest to game developers but also more broadly can measure brain activity patterns,” he said.
Boas, who is Franceschini’s husband, said fNIRS often works in conjunction with other technologies, such as diffuse correlation spectroscopy and laser speckle contrast imaging, which capture the temporal and spatial resolution of various biomarkers in the brain, respectively.
In his own lab, Boas has used fNIRS to monitor brain activity during a variety of prescribed tasks, such as during surgery or mental exercises, in which a cap equipped with optodes is placed on a subject’s head.
“Right now, there is race to see who can get the next fNIRS device to market,” he said.
References
1. K-C. Wu et al. (2022). Open-source FlexNIRS: a low-cost, wireless and wearable cerebral health tracker. NeuroImage, Vol. 256, No. 119216.
2. H. Ban et al. (2022). Kernel Flow: a high channel count scalable time-domain functional near-infrared spectroscopy system. J Biomed Optics, Vol. 27, No. 7.