Every photon does count, not only in research but also, increasingly, in business. Single-photon detection is at the heart of quantum communications, which offers absolute protection against eavesdropping. The technology is moving out of test beds and is being employed in some important settings.
For example, quantum equipment from Geneva-based id Quantique SA encrypted network traffic for the federal elections in Switzerland in 2007, said CEO Grégoire Ribordy. “That was, to my knowledge, the first application of quantum cryptography with real data – a real network and a critical application.”
However, quantum communications is only the start of what single-photon detectors might deliver. Quantum computers, which are based on the same technology, could someday tackle problems that are impossible for today’s machines to solve.

A 1064-nm single-photon detector system based on an indium phosphide detector is shown. Courtesy of Princeton Lightwave Inc.
It all begins with individual photons and the right detector. That explains why researchers and vendors are scrambling to develop devices that meet the demands of quantum information processing.
Counting single photons for fun and profit
Single-photon detection plays a key role in quantum information processing because data is encoded in the polarization or in some quantum-related characteristic of photons. The speed and accuracy of photon detection play an important role in overall system performance. Although single-photon sources still need development, the bottleneck currently lies in the detectors.
Philip Walther, a quantum information researcher at the University of Vienna in Austria, noted that state-of-the-art silicon avalanche photodetectors are about 40 percent efficient at typically used wavelengths, such as visible and near-infrared. The devices were employed by researchers from the university and elsewhere to do quantum key distribution through the air over a distance of 144 km.
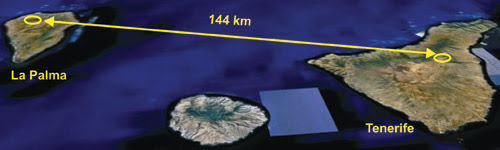
Quantum key distribution has been done between the islands of Tenerife and La Palma in the Canary Islands. Courtesy of Philip Walther, University of Vienna.
The investigators successfully spanned this distance by increasing the intensity of the source, thereby overcoming significant transmission losses. “However, more efficient detectors would also significantly boost the count rates,” Walther said.
The group’s accomplishment opens the door to securely distributing encryption keys worldwide via quantum technology and satellites. Members of the same group experimentally verified the feasibility of a quantum channel between space and Earth, reporting on a successful test in the March issue of the New Journal of Physics.
Commercial quantum communication is focused on telecom wavelengths – up around 1500 nm – partly because of the availability of high-quality lasers at these wavelengths and because the existing telecom optical fiber is optimized for these wavelengths.
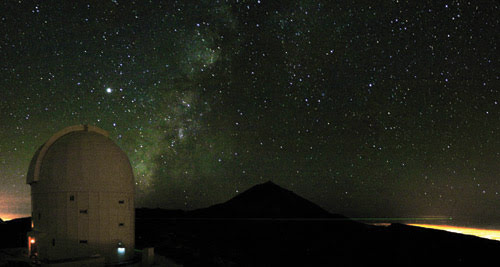
With lasers, telescopes and single-photon detectors, researchers sent quantum-encoded information through the air across a span of 144 km. Courtesy of Philip Walther, University of Vienna.
The problem is the speed of available avalanche photodiodes, which are made out of indium gallium arsenide, indium phosphide or other so-called three-five semiconductors. When one or more photons arrive, the result is a flood of electrons, as many as a million for a single photon. That burst and its aftermath limit the speed of detection, said id Quantique’s Ribordy. “You have these after-pulsing phenomena, which forces you to apply a dead time.”
Michael LaGasse is vice president of engineering for New York-based MagiQ Technologies, a company that makes quantum cryptography products. He noted that the current devices work and can be used in a production environment, as evidenced by a demonstration done in October in Vienna.
The trial consisted of a six-node network and eight intermediary links of up to 82 km. Encryption keys were generated and distributed with quantum cryptography over standard telecommunication fibers and in free space along a line of sight between two telescopes. Various quantum technological devices for generating keys were integrated into the network over standardized interfaces.
The avalanche photodiodes used in the current crop of commercial quantum products often are specially packaged and cooled, the latter being done to reduce noise. Because they must register single photons, they are operated in such a way that the attainable speeds are much lower than the devices are capable of under other conditions.
“Typically, people run these so they don’t see significant amounts of after-pulsing events, and that brings you down to a much lower rate than the gigahertz rate that they’re designed for,” LaGasse said.
Consequently, researchers and vendors are looking for ways around this problem. One possibility would be to change the electronics, which could allow a faster readout. Another would be to change the material or basic technology used.
Decreasing dark current
One vendor working to improve single-photon detectors is Princeton Lightwave Inc. of Cranbury, N.J. Bruce Nyman, the company’s vice president of systems solutions, noted that the firm makes only compound semiconductor detectors, focusing on indium phosphide ones that operate in the infrared. In response to demands from the quantum information processing community, the company has been improving device performance.
Part of the work has been aimed at device packaging. To achieve the necessary performance, the detectors typically are cooled to –60 °C. As a result, condensation can be a problem. To avoid this, the company seals the detectors in a hermetic package that keeps out moisture.
Innovations in device fabrication and design have led to other recent improvements, according to Nyman. “We’ve decreased the dark count, which is a key parameter for system design. We’ve been able to cut that by at least an order of magnitude.”
In conjunction with NASA, the company also has developed an indium phosphide-based device capable of single-photon counting in the 950- to 1100-nm range. It offers a detection efficiency of 20 percent at 1064 nm, which Nyman noted is much higher than the few percent available at the same wavelength from current silicon-based devices.
One possible application for the device is in lidar. Being able to detect single returning photons would extend the lidar’s scanning distance.
A cool, new technology
Improved detectors also are under development in laboratories around the world. Some are enhancements to and innovations of existing approaches. Some take a totally different tack, such as by exploiting superconductivity. Commercial concerns are interested in these techniques, although the expectation is that it will be three or more years before these detectors will become products.
Sae Woo Nam, a physicist at the National Institute of Standards and Technology (NIST) in Boulder, Colo., headed a team that reported in the March 3 issue of Optics Express that it had produced about a 95 percent efficiency in counting near-infrared photons. The exact figure is a bit of an unknown because there are no accepted ways to measure the efficiency with sufficiently high accuracy.
The team’s approach is to do away with a semiconductor and instead use a superconducting metal. With a semiconductor, an incoming photon must make an electron-hole pair to be detected. However, there are many ways in which the excitation from a photon can be dissipated, not all of which result in an electron-hole pair measurement. The situation is different in a metal.
“In the metal, what we’re doing is measuring the temperature rise,” Nam explained. “It’s a conceptually simpler device.”
To make that measurement, the researchers used a superconducting transition edge sensor made of tungsten fabricated in an optical structure and optimized to absorb at 1310 and 1550 nm. They cooled the detector, which measured 25 µm on a side and was about 20 nm thick, to 100 mK – just below its superconducting transition temperature. When a photon was absorbed by the metal, it elevated the temperature, causing a detectable change in resistance.
After having achieved this efficiency milestone, the investigators are working on additional improvements. They hope to increase the detector’s speed but are limited in this by the material itself. Before their system can detect another photon, it must cool back down below the transition temperature. A different material with a faster thermal time constant would yield a faster device. Nam said a tenfold or more increase in speed is possible.
The group also is also working to improve efficiency even more. Better performance in this regard could be critical for quantum computing. In communications, a dropped photon can be re-sent and so the penalty for inefficiency is a slower data rate. In computing, a missing photon could mean that a program runs incorrectly and that the results are wrong. This is particularly true for what is known as one-way quantum computing, in which the transmission itself is the program and data. A series of consecutive measurements define and run the program.
Detectors intended for generalized quantum information processing have another requirement. Ideally, they must be able to tell how many photons arrive in a given pulse. This number-resolving capability is not found in current photodetectors, which operate in Geiger mode. Whether one or many photons arrive at one time, they report the equivalent of a single click.
Nam said the group’s superconducting transition-edge sensors can meet this need. “It offers both high detection efficiency and number resolving.”

A transition-edge sensor detects and counts photons through the change in temperature of a superconducting metal, in this case tungsten. The schematic on the right shows the process, while actual sensors are shown on the left. Courtesy of Sae Woo Nam, NIST.
Detection by wires
One of the most promising new single-photon-counting technologies is on the other end of the spectrum, efficiency-wise, from transition-edge sensors. These are superconducting nanowires made out of niobium nitride, and they currently have a typical practical efficiency of about 1 percent at 1550 nm. Part of the reason for the low efficiency is that these detectors consist of 100-nm-wide stripes separated from one another by about the same distance. The stripes meander up and down to cover an area that measures about 10 ?m on a side. It is difficult to couple the fiber to the detector, however, so some photons are lost.
But that is not the entire reason why the efficiency is so low. Robert H. Hadfield, an assistant professor of physics at Heriot-Watt University in Edinburgh, UK, who investigates superconducting nanowires, noted that the efficiency problem also is the result of the ultrathin material, which is in a film only 4 nm thick. “It’s semitransparent. A lot of the light simply goes through.”
A group led by MIT professor Karl Berggren demonstrated one possible solution to this problem. It placed a reflector behind the nanowire and implemented other changes, thereby achieving detection efficiencies of about 50 percent at 1550 nm. Researchers at other locations also are working on improving efficiency through various geometries and optical arrangements.
What nanowires offer over their competition are very low dark counts and very good timing resolution. The combination translates into high data rates – fast enough to overcome low efficiency. These detectors also have to be cooled to only about 4 K, which now can be achieved in fairly inexpensive closed-cycle refrigerators.
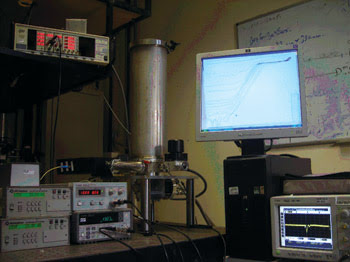
Shown here is a superconducting nanowire single-photon-detector system based on a closed-cycle refrigerator. Courtesy of Robert H. Hadfield, Heriot-Watt University.
That latter development makes it possible to place several detectors into one setup, cutting down on the cost of implementing a nanowire single-photon-detection system. That’s good news for quantum communications, quantum computing and other users of single-photon counters.
Another bit of good news is Hadfield’s own research focus, which promises to help bring these detectors out of the lab and into everyday use. “I’m trying to integrate the detectors into practical systems.”