Take the Low and High Roads to Imaging Success
Michael R. Feinberg and Paul Bierden, Boston Micromachines Corp.
Researchers worldwide have leveraged advances
in microelectromechanical systems (MEMS) mirror technology by using adaptive optics
to correct for wavefront aberrations caused by distortion. Now, the next generation
of adaptive optics systems is using dual deformable mirror configurations to effectively
compensate for a variety of wavefront aberrations. Researchers have opened possibilities
in ground-based astronomy and biological imaging that previously were unimaginable.
The right mirror for the job
Deformable mirrors are advanced wavefront control devices that
can change shape to correct a distorted incident wavefront. The fundamental specifications
for deformable mirror systems are resolution, spatial frequency, speed, stroke and
surface finish.
The resolution is determined by the number of actuators in the
mirror array, which ranges from 19 for an entry-level membrane-based device to more
than 4000 for a MEMS deformable mirror. Spatial resolution is a measure of how complex
a wavefront the deformable mirror can correct. This is determined by the number
of actuators that control the shape of the mirror and by the mechanical coupling
between adjacent actuators. Speed is based on the architecture and material properties
of the deformable mirror. Finally, stroke is a measure of maximum actuator deflection.
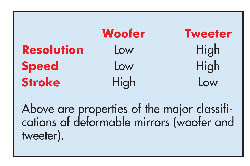
As the technology stands today, mirrors are most proficient at
either low-resolution, low-speed and high-stroke operation, or high-resolution,
high-speed and low-stroke operation, identified as “woofer” and “tweeter,”
respectively (see table). It is this tradeoff that necessitates a next-generation
approach to adaptive optics wavefront correction.
To achieve a higher degree of wavefront correction, newer adaptive
optics systems are using both a woofer and tweeter style of deformable mirror. The
woofer/tweeter dual-mirror configuration allows for better compensation of large-variance,
high-spatial-frequency phase distortion. A simplified layout of such a system is
shown in Figure 1.
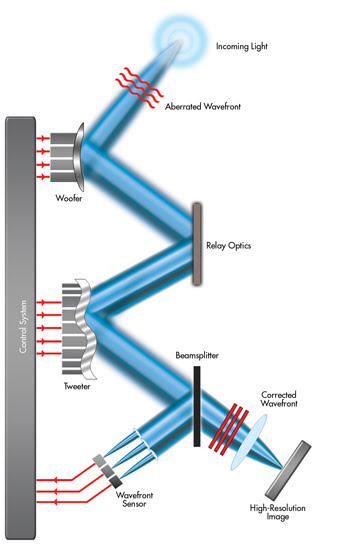
Figure 1. Shown is a simplified layout of a typical woofer/tweeter
adaptive optics system. In this configuration, incoming light is reflected off the
woofer and tweeter mirrors, which are managed by a single control system. The deflection
of each mirror is determined by an algorithm that calculates the optimal mirror
shapes to compensate for aberrations as measured by a wavefront sensor.
Low-order optical aberrations are the most common and are corrected
using a high-stroke, low-resolution mirror (the woofer). This can be accomplished
using a membrane-type mirror with a limited number of actuators. High-order aberrations
are more complex and require more precision. Most microscopy, vision science and
laser shaping applications require 1 to 4 μm of stroke to correct for these
aberrations, which is achievable with a high-resolution mirror (the tweeter).
One mirror that can fill the role of the tweeter is a MEMS deformable,
which consists of a mirror membrane – either continuous or segmented –
supported by an underlying actuator array. Each actuator in the array can be deflected
by electrostatic actuation to achieve the desired pattern of deformation.
Scientists have been developing dual deformable mirror woofer/tweeter
systems to deploy in adaptive optics applications for retinal imaging and astronomy.
Vision science applications
Leading vision scientists and ophthalmologists believe that the
human retina will be a window into human health. Being able to visualize the retina
at a cellular level gives researchers the ability to study vasculature and photoreceptor
properties and holds promise for earlier diagnosis of the “big three”
eye diseases: glaucoma, diabetic retinopathy and age-related macular degeneration.
Wavefront distortions generated in the eye itself prevent generation
of useful high-resolution images without the use of adaptive optics, which corrects
distortions introduced by the cornea, crystalline lens and vitreous humor. Adaptive
optics enables increased contrast levels and unprecedented retinal resolution levels.
In retinal imaging with an adaptive optics scanning laser ophthalmoscope
(AOSLO), a dual deformable mirror approach could be used because of the large individual
differences in defocus and astigmatism in humans. For the first time, researchers
can see critical detail within the retina and can detect changes in the eye significantly
earlier than with current diagnostic tools. Earlier detection can enable early treatment
that could slow the progression of eye disease – or even prevent it.
Steps are being taken to detect disease through the use of an
AOSLO system at Indiana University Bloomington, where researchers Stephen A. Burns,
Weiyao Zou and Xiaofeng Qi have developed a real-time zonal control algorithm that
uses wavefront slope measurements from a single Shack-Hartmann wavefront sensor
to generate control signals for two deformable mirrors.
The procedure will be used to implement a woofer/tweeter dual
deformable mirror AOSLO system for in vivo human retinal imaging with a 140-actuator
deformable mirror (maximum stroke, 3.5 µm) and a 52-actuator magnetic deformable
mirror (maximum stroke, 50 µm). The low-stroke deformable mirror is the tweeter,
for correcting the high-order aberrations; the high-stroke deformable mirror is
the woofer, for correcting the low-order aberrations. The dual-mirror system effectively
removed aberrations in the eye, thereby generating clear images that will be used
to study the progression of eye disease in live patients.
Astronomy applications
Adaptive optics also is commonly used on telescopes to remove
the effects of atmospheric distortion. When light from a star or another astronomical
object enters the Earth’s atmosphere, turbulence distorts the light in various
ways, including blurring images. An adaptive optics system tries to correct this
by using a wavefront sensor that takes some of the astronomical light for analysis,
a deformable mirror that lies in the optical path and a computer that receives input
from the sensor. The sensor measures the distortions that the atmosphere has introduced
on the timescale of a few milliseconds, and the computer calculates the optimal
mirror shape to correct them. The surface of the deformable mirror is reshaped accordingly.
One system under construction that will use a woofer/tweeter design
is the Gemini Planet Imager, a next-generation adaptive optics instrument being
built for the Gemini telescope by a consortium of US and Canadian institutions.
Funded by the Gemini Observatory, a partnership of seven nations, the group’s
goal is to image extrasolar planets orbiting nearby stars using a dual deformable
mirror system. The Gemini system’s low-actuator-count woofer reduces the residual
wavefront error to a level controllable by the finer tweeter, a MEMS deformable
mirror with 4096 active elements. First light is projected to be recorded in early
2011.
A second example is the PALM-3000, a high-precision upgrade to
the Palomar Adaptive Optics System on the 5.1-m Hale Telescope at Palomar Observatory
in Palomar Mountain, Calif. It will use its existing deformable mirror (241 active
actuators) as the woofer and a new high-actuator-count deformable mirror (3388 active
actuators) as its tweeter. As with the Gemini Planet Imager, first light is projected
to be in early 2011.
Dual-mirror control
Two distinct methods for control of dual deformable mirror woofer/tweeter
systems have been developed – serial and parallel. Using a serial approach,
the aberration is corrected by the woofer first, and the resulting image is sequentially
corrected by the tweeter. This is a “best effort” approach and is currently
taking a backseat to a more real-time parallel approach. With improved algorithms
and faster processing speeds, most applications now take advantage of parallel correction
methods. To do this, the algorithm sorts the wavefront aberrations into two groups
– one for the woofer correction and one for the tweeter correction –
in real time.
A recent algorithm developed for vision science has come from
Chaohong Li, Nripun Sredar, Hope Queener, Kevin M. Ivers and Jason Porter at the
University of Houston’s College of Optometry in Texas. The success of this
technique is shown in Figure 2, which depicts a high-resolution image of photoreceptors
in the retina.
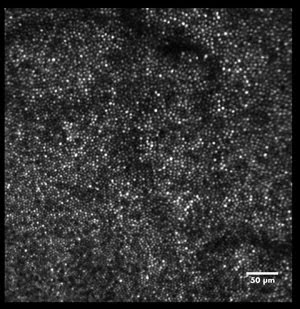
Figure 2. This image
shows individual photoreceptors in the retina using an adaptive optics scanning
laser ophthalmoscope with woofer/tweeter optical architecture. Courtesy of Kevin
M. Ivers, Chaohong Li and Jason Porter, University of Houston College of Optometry.
For astronomical applications, previous work has been done as
part of the “Woofer Tweeter Experiment” at the University of Victoria
in British Columbia, Canada, and is currently proceeding for the Palomar Observatory’s
PALM-3000 adaptive optics upgrade.
Adaptive optics continues to evolve to provide clarity. Using
dual deformable mirror devices, biological imaging researchers can look deeper in
vivo and astronomers can obtain higher-resolution images of celestial objects, enabling
further research into the behavior of other solar systems. The woofer/tweeter optical
architecture provides continuous improvement to correct wavefront aberrations and
promises to lead to new discoveries in all types of imaging applications.
Meet the authors
Paul Bierden is president and CEO of Boston Micromachines Corp.
in Cambridge, Mass. Michael R. Feinberg is the company’s director of product
marketing; e-mail: [email protected].