John Cowley for ColdQuanta Inc.
Bringing cold atoms to the classroom gives undergraduate students a hands-on way to learn
about physics – and could inspire lifetime careers using photonics to manipulate
atoms.
Bringing cutting-edge physics into the undergraduate
classroom is not always easy. “Cold atoms” (<0.0003 K) were first
produced in the late 1980s, but creating the right conditions for them still requires
research specialists in advanced physics laboratories. Re-creating the original
experiment that led to the 1997 Nobel Prize in physics (which went to Steven Chu,
Claude Cohen-Tannoudji and William D. Phillips) is demanding: Expertise with high
vacuums (10–7 torr), atom sources, ion pumps, magnetic fields, and optical
and laser alignment is required.
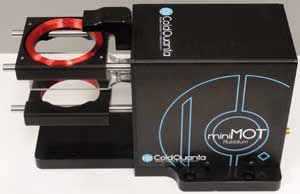
Figure 1. View of the miniMOT package showing the evacuated glass chamber located between the magnetic coils. Courtesy of ColdQuanta Inc.
Within the field of experimental physics, researchers are increasingly
aware of the importance of intensive experience with cold atoms. Now, a three-week
project allows assistant professor Dr. Heather Lewandowski’s senior physics
students at the University of Colorado at Boulder to get that experience through
cooling and trapping clouds of rubidium atoms. Luckily, they don’t spend most
of that time setting up or finely tuning their equipment; instead, they spend it
on their experiments, thanks to a preassembled, prealigned setup called the miniMOT
kit.
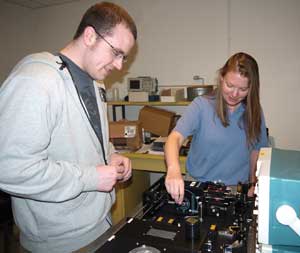
Figure 2. Dr. Heather Lewandowski and a student work with the miniMOT
at the University of Colorado at Boulder.
MOT, which stands for magneto-optical trap, was coined by the
physics research community. The miniMOT kit, which was developed by Boulder startup
ColdQuanta Inc., allows undergrad students to jump over setup hurdles, right into
the study of cold atoms. Rainer Kunz, ColdQuanta’s CEO, predicts that the
device will become as indispensable to college physics laboratories as the oscilloscope
is today.
The central feature within the miniMOT package is an evacuated
glass experimental chamber that allows laser-beam access along three mutually perpendicular
axes. This geometry is critical for laser cooling of target atoms within the chamber.
The chamber is sealed and shipped with the required vacuum, which means that users
need no vacuum experience or equipment.
The system uses rubidium atoms, which respond to general-purpose
780-nm lasers. An active rubidium dispenser resides within the evacuated chamber
to make it easy to inject the material for experiments. The chamber also holds a
nonevaporable getter and an ion pump to maintain the vacuum. All of the electronic
circuits to power the ion pump and the rubidium source are integrated into the package,
eliminating the need for external ion-pump controllers and current supplies for
the vacuum system. The design includes the hardware needed to mount optics (mirrors
and wave plates) along the vertical axis. The enclosure’s footprint is <250
cm2 (38 in2) and volume is ~3.1 liters (~190 in3). For the hardware included with the miniMOT kit, see “Just add lasers” on the right.
Some instructors may be concerned that the convenience provided
by prealigned kits could rob students of the learning experience of fine-tuning
the experimental setups associated with research in atomic optics. That is a trade-off
decision the instructor must make: Do I offer a class that covers many aspects of
experimental physics in one semester or one that focuses on fewer experiments and
provides time for preparatory alignments? For those who choose the latter, students
can remove and restore the optics or use the nonkit version of the miniMOT.
All kinds of experiments
The following types of experiments with cold atoms are now accessible
to a wide range of students and researchers through use of the miniMOT kit:
• Demonstrating laser cooling. Students can go from classroom
theories of how lasers can reduce atomic energies to actually accomplishing laser
cooling in practice. They will see how a properly arranged laser configuration can
create a “cloud,” faintly visible in the near-infrared, of cold atoms
at the intersection of the beams. They also can experience how a secondary, parallel
set of laser beams, detuned about 0.01 nm, can be used to re-pump errant atoms to
maintain the trapped cloud.
• Demonstrating magnetic trapping. Laser cooling reduces
the velocity of the atoms, but the further confinement (trapping) of the atoms within
a small region is effected by applying a tailored magnetic field. Students can energize
two coils on the miniMOT’s supporting rods to establish an anti-Helmholtz
magnetic configuration, which creates a small region of zero magnetic field within
the glass chamber where a cloud of cold atoms will become trapped (see Figure 3).
The zero-field region forms a stable location for the atoms in conjunction with
their spin magnetic moments.
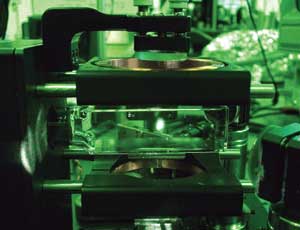
Figure 3. View of cold-atom cloud (central bright spot) trapped in the zero-field region of the two coils.
• Measuring atom number. Once an atomic cloud has been created
and confined, students can experimentally estimate the number of atoms in the cloud.
The brightness of the ensemble of photons, continuously re-radiated by the trapped
rubidium atoms, is related to the number of atoms. By using a lens and a CCD sensor
suitable for detecting the near-infrared radiation, a sector of the sphere of radiation
can be imaged and its brightness measured – raw data needed to estimate the
number of atoms. This experiment also trains students to account for the many error
sources inherent in this type of research.
• Measuring the temperature of the atom cloud, which is proportional
to the average of the square of the atoms’ velocities. To deduce temperature,
the average velocity can be revealed by disabling the magneto-optical trap and observing
how quickly the atomic cloud expands. Data for cloud size versus time can be taken
by sequential CCD images of the cloud’s shadow or by sequential images of
the cloud fluorescing in response to brief laser pulses.
• More advanced research. Besides the above examples, the
miniMOT package permits advanced students and researchers to conduct more demanding
experiments in atomic physics. These include applications to quantum entanglement,
quantum optics, atomic clocks and electromagnetically induced transparency, or “slow
light.”
Dr. Andrew M.C. Dawes, assistant professor of physics at Pacific University in Forest Grove, Ore., has used the miniMOT to form a long, narrow (anisotropic) cloud of cold atoms to study the interaction of photons with the rubidium atoms.
“By sending light along the long axis of the cloud, the
light-matter interaction region is maximized,” he said. “These so-called
anisotropic atom clouds combine the benefits of cold atomic media with long cloud
geometry to achieve strong light-matter coupling. The anisotropic atom cloud is
a promising medium for experimental tests of all-optical information processing.”
He noted that the setup also enables experiments such as nonlinear wave mixing in
cold atoms, tests of light-guiding in the anisotropic MOT and transverse optical
pattern formation.
With the use of additional hardware, future experiments incorporating
the miniMOT will produce even colder atomic clouds (20 μK or colder) using
sub-Doppler laser cooling.
Lab guides to come
To promote cold-atom research in the undergraduate physics curriculum,
the University of Colorado’s Lewandowski is developing instructor and student
lab guides for working with the miniMOT kit. These free guides, supported by a grant
from the National Science Foundation, should be available in late 2011.
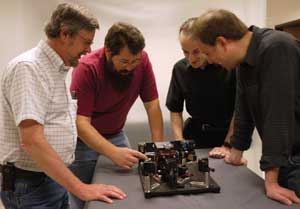
Figure 4. View of technical team with the miniMOT.
Importantly, the guides will help instructors evaluate how well
the students achieve the educational goals. They are designed to confirm that the
students have learned the appropriate laboratory skills and physics principles in
the process of carrying out their experiments.
In recent years, the photonics industry has searched for ways
to inspire students to enter photonics-related careers, in part because of a shortage
of qualified job applicants. Hands-on experience at the undergraduate level could
help do just that.
Meet the author
John Cowley is a retired aerospace engineer and freelance writer
living in Boulder, Colo.
Just add lasers (and a few other things)
The miniMOT is available preassembled and prealigned,
with most of the additional hardware needed to produce cold-atom clouds in the undergraduate
setting.
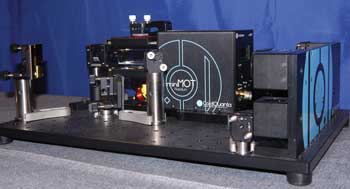
Side view of the miniMOT kit
The miniMOT kit includes:
• Optics for manipulating the impinging laser beams (periscope, beamsplitting
and polarization components).
• Mirrors to facilitate alignment of the laser beams for
atom cooling.
• Coils to create the trapping magnetic field.
• A small black-and-white CCD camera for imaging the trapped cold-atom cloud.
• A 15 x 24-in. optics breadboard, upon which the supporting components are mounted and prealigned.
The user need only provide the lasers, laser interface, video
monitor and power supply for the magnetic coils.