For any job, there is the right tool. But understanding how to evaluate your equipment and recognize its limitations could be the key to raising the standard of the science you carry out.
The technology surrounding microscopy systems is evolving at an astounding and sometimes overwhelming pace. For example, light sources have advanced from bulbs with a lifetime of a few thousand hours to LEDs or lasers with significantly longer lifetimes. These light technologies are available at higher intensities and at a wider variety of emission wavelengths.
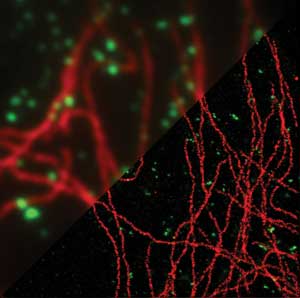
The wide-field superresolution technique GSDIM (ground state depletion followed by individual molecule return) is a localization microscopy method that resolves details as small as 20 nm. The Leica SR GSD was launched in 2011 and recently given the R&D 100 Award. Courtesy of Professor Dr. Stefan Hell, Max Planck Institute for Biophysical Chemistry, Göttingen, Germany.
Similarly, camera technology continues to push boundaries. Increased speeds in EMCCD (electron multiplying charge-coupled device) cameras directly benefit emerging superresolution applications.
Keeping up with technological advances could improve the quality of data extracted from an imaging system, but often microscopy users consider a new system only when they find that their existing equipment falls short.
“Researchers generally review and evaluate microscope instrumentation as their research dictates,” said Mark Clymer, product manager of the Core Microscopes Scientific Equipment Group at Olympus America Inc. of Center Valley, Pa. “As their research evolves, they may find that their current microscope system cannot adequately capture the biology being studied – perhaps the system or detector (i.e., camera) is too slow; perhaps the wavelengths needed for the probes aren’t sufficient; perhaps they require greater automation than is afforded by their current system.”
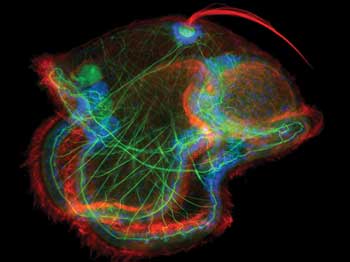
Wide-field image of a pilidium larvae of the Nemertean ribbon worm, Cerebratulus lacteus, stained for F-actin (green; phalloidin), Acetylated tubulin (red) and DAPI (blue; nuclei). Imaged with Zeiss Axio Imager. Courtesy of Marine Biological Laboratory, Woods Hole, and Development.
Application, future-proofing, support
The most important properties to consider when purchasing a microscopy system will depend on its intended application; after all, the forthcoming experiment determines the required objectives, filters, imaging detector, illumination source and imaging technique.
Defining the requirements of the application is crucial to making a final decision. For example, if the researcher is looking at bright-field fixed-cell samples, the key requirements may be a full white-light spectrum illumination source and a detector that represents the colors of the sample accurately – with sensitivity and throughput being lower priorities.
Conversely, if the researcher is conducting single-molecule fluorescence studies, the requirements are more demanding regarding the sensitivity of the detector as well as the use of appropriate filters and the throughput of the optics. The light source spectrum and intensity requirements are also dependent upon the sample.
But purely technological topics are not the only important ones; also critical is whether the microscope will support the application efficiently, as Markus Wiederspahn at Carl Zeiss Microscopy GmbH in Jena, Germany, pointed out.
“Usability, modularity, upgradability and support are key to ensure and maintain top-level performance of the instrument. Handshakes between technologies (detectors) are important – i.e., an open software environment ensures integration and easy handling to correlate the results,” he said. “Experienced application specialists from the microscope supplier will be able to help with this question.”
Most manufacturers acknowledge that once the application is addressed, then service support and future-proofing are the next crucial factors.
“It is said that before making a purchase, customers look mostly at specifications and performance,” said Rachit Mohindra, product manager at Photometrics of Tucson, Ariz. “After a purchase, customers are significantly more interested in the service and support offered by the supplier. This is a very commonly overlooked factor when making a purchase, and it can have significant effects on the ability of the customer to maximize their research potential on a given microscopy system.”
Clymer at Olympus agrees. “It can be difficult to predict how your research needs evolve and therefore how the microscope system will need to accommodate those changes,” he said. “Important considerations, and often overlooked, are the support and service provided by the sales representatives and the manufacturer. Are they there for you? Do they respond to your questions quickly? Do they help you get the most out of your microscope?”
Other important considerations to keep in mind include the number of user groups, according to Dr. Tanjef Szellas, head of product management of confocal microscopy at Leica Microsystems in Wetzlar, Germany. If multiple research groups will be using a single microscopy system, flexibility is crucial. The expertise of users could also come into play, meaning ease of use must be considered.
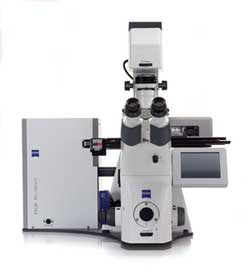
The PALM MicroBeam by Carl Zeiss Microscopy. The precise and contact-free laser microdissection system isolates single cell populations from heterogeneous samples. This opens up new prospects for molecular research. Courtesy of Carl Zeiss Microscopy.
But Clymer sounds a note of caution: Trying to find a single microscope that can do everything is usually impossible.
“A microscopy system built up to try to do everything is like a hybrid bicycle – sure, it’s functional, but a mountain bike will always outperform the hybrid on the trails, and a road bike will outperform it on the roads,” Clymer said. “Therefore, researchers will purchase multiple microscopy systems, each of which has been configured to perform specific specialized tasks.”
Microscopy systems: Top considerations
• Performance/application demands
• Ability to upgrade the system later (future-proof investment)
• Single user group or multiple research groups (flexibility)
• Expertise of users (ease of use)
• Efforts for system maintenance/support
• System sensitivity
• Recording speed (important for live-cell imaging)
• Versatility (modularity)
• Stability (long-term measurements, no software crashes)
• Optical performance (field flatness, color correction)
• Handshakes between technologies
• Robustness
• Ergonomics
“With higher demands on microscopes, it is important to get the best specific solution for your application rather than a ‘one size fits all’ solution,” said Wiederspahn at Carl Zeiss Microscopy. “Companies with broad product [portfolios] are the best choice to ensure the consulting phase is not biased towards the ‘what we have in our basket’ solution.”
To buy new, or not?
With modular components available from many manufacturers, users often can upgrade their microscopes rather than replace them completely.
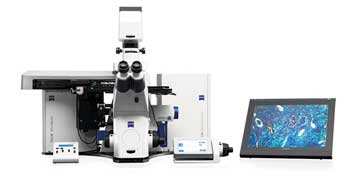
The PALM CombiSystem by Carl Zeiss Microscopy. Dedicated for contact-free micromanipulation, this modular instrument combines the benefits of laser microdissection with optical trapping. Courtesy of Carl Zeiss Microscopy.
As the system is used, there are parts that may age or decay over time; these include light sources (metal halide bulbs, mercury bulbs) as well as objects in the optical path that may have to be replaced or updated (filters, dirty mirrors, objectives) to maximize the quality of experimental results.
And there are numerous third-party suppliers who produce aftermarket products that can be used to upgrade the microscope (i.e., stages, light sources and illumination control).
“Through the addition of accessories, most research-level microscopes can grow with your research,” Clymer said. “Along with the variety of standard motorized, coded and manual components and accessories, the new Olympus IX83 has an available ‘breadboard’ insert that enables researchers to create their own custom access for a wide assortment of techniques including optical trapping, TIRF [total internal reflection fluorescence], FRAP [fluorescence recovery after photobleaching], etc.”
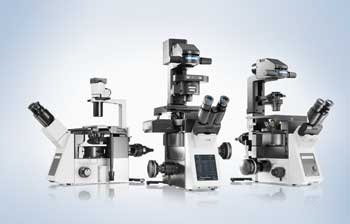
The Olympus IX3 line of inverted research microscopes includes the IX83, a fully automated high-end research microscope platform; the IX73, which can be configured in manual, semimotorized or motorized versions; and the IX53 for quick and efficient routine examination of multiple cell or tissue samples. The IX83 and IX73 are designed with an open multilevel frame that can hold one or two optical decks, allowing for insertion of various optical components. The decks make the microscopes truly modular, enabling rapid plug-and-play insertion of magnification changers, filter turrets, a right-side port or other options. Changing equipment and options is as easy as sliding out one deck insert and sliding in another, and both decks open out like file cabinet drawers for easy accessibility. The large open available space also is compatible with user-designed modules. Courtesy of Olympus America Inc.
Investing for the future: Seen and unseen
It is useful to plan for future experimental needs; however, looking for experimental hardware and software that go too far beyond current needs could mean that you risk surpassing your budget.
“If future requirements are purchased too far in advance, the system may not be used to its full potential due to future technology developments that render the current latest and greatest obsolete,” said James Joubert, applications scientist at Photometrics. “Many times the requirements definition process becomes a matter of making a list of needs versus wants and then balancing these requirements with the available budget.”
Significant progress has been made recently with superresolution systems, which allow researchers to achieve resolutions as low as 5 nm (depending upon experimental conditions). These statistical localization algorithm-based methods are pushing the limits of physics and detection capabilities of current microscopy systems.
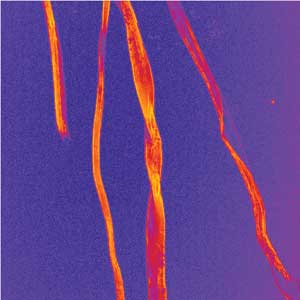
CARS (coherent anti-Stokes Raman spectroscopy) image of cellulose fibers. CARS microscopy is a technique that generates images based on the vibrational signatures of molecules. This imaging method does not require labeling, yet molecular-specific information can be obtained from a range of important biomolecular compounds. Leica Microsystems recently launched the Leica TCS SP8 CARS. Courtesy of professor Eric Potma, University of California, Irvine.
While superresolution is of growing importance in fields such as neuroscience, Szellas pointed out that label-free imaging like coherent anti-Stokes Raman spectroscopy (CARS) is becoming more relevant for health care-related science, such as lipid imaging.
“The requirements are so diverse. Leica Microsystems aims to set up the systems in such a way that a scientist is able to adapt easily to any upcoming new field of application,” he said.
To safeguard your investment, an element of future-proofing is prudent, making modularity and upgradability important considerations. But since you can’t guarantee what the future will bring, it is best to buy for right now, not for what might be right in the future.
“The only time that the potential for a breakthrough method should impact your purchasing decision is if that method is immediately applicable and relevant to your research,” Clymer said. “Otherwise, it often proves wiser to allow manufacturers to refine the method and delivery approach before jumping in. Consider also that most of the techniques have been years in the making, often invented on older microscope models; it takes time to develop integratable components, accessories and software control.”