Alana Achterkirchen, Rad-Icon Imaging, a Division of Teledyne Dalsa
There still are many unanswered questions in high-energy astrophysics: What is happening in the universe to produce so much gamma-ray energy? What happens to the surrounding environment near solar flares? Some of the answers could come from studying gamma-ray-producing phenomena.
Scientists still are studying many of the universe’s most exotic and beautiful phenomena. Recent research has highlighted supernova explosions, black holes, merging neutron stars and solar flares, all of which generate gamma-ray radiation. Gamma rays are the most energetic form of light – billions of times more energetic than the type of light visible to our eyes – and produce the most energy of any other wave in the electromagnetic spectrum, according to NASA. The progression from designing, testing and implementing gamma-ray instrumentation requires long, iterative cycles of development – it’s hardly a race to the sun.
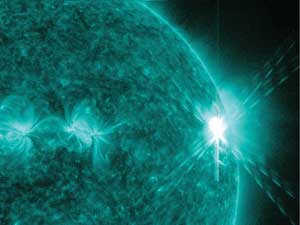
An X-class solar flare, as captured by NASA’s Solar Dynamics Observatory in an unrelated project. Courtesy of NASA.
To study solar flares in the wide energy band of the electromagnetic spectrum ranging from UV to high-energy gamma rays (2000 MeV), three Roentgen telescopes were developed by Dr. A. Raghu Rao of the astronomy and astrophysics department at Tata Institute of Fundamental Research in Mumbai, India, and a team of Indian researchers. The instruments were constructed by a collaboration of the institute, the Vikram Sarabhai Space Centre and the Indian Centre for Space Physics.
Astrophysics imaging is a highly specialized field, where the instruments are mainly custom-designed, but the design of these Roentgen telescopes proves that highly effective instruments can indeed be designed from component technologies.
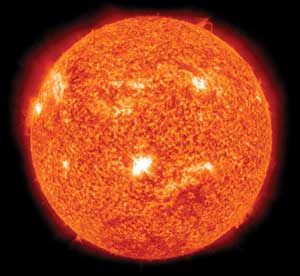
A strong solar flare, recorded by NASA’s Solar Dynamics Observatory in an unrelated project. Courtesy of NASA/SDO.
CMOS sensors for astronomy
CMOS x-ray sensors and cameras generally are designed for industrial and medical imaging applications, but there is a precedent for using this technology in aeronautics research: A CMOS x-ray camera from Rad-icon Imaging of Sunnyvale, Calif., a division of Teledyne Dalsa of Waterloo, Ontario, Canada, helped Dr. Upendra D. Desai of NASA Goddard perform ground-based gamma-ray astronomy in 2001. Desai demonstrated that cost-efficient CMOS technology provides higher resolution than standard gamma-ray detector technologies, such as wire chambers or cadmium zinc telluride (CZT) detectors. He pioneered a technique to cool the camera to –20 °C, enabling long integration times, and used a Fresnel zone plate to focus gamma-ray images onto the camera to achieve his imaging requirements.
When Rao designed his solid-state RT-2 (RT is short for Roentgen telescope), he needed to capture high-resolution images of the solar flares in hard x-rays. The RT-2 CMOS/CZT telescope incorporates one RadEye1 CMOS detector from Rad-icon Imaging and three CZT detector modules from Orbotech Medical Solutions Ltd. of Rehoboth, Israel, a subsidiary of GE Medical Systems Israel Ltd. of Haifa.
Rao leveraged the architectures of CMOS and CZT and tuned the array for astrophysics imaging. The 2 x 2 array captures high-resolution color images with very fine energy discrimination capabilities.
Rad-icon’s CMOS x-ray detectors offer resolution from 22 to 100 µm with no energy discrimination; CZT detectors have a strong energy discrimination that can pinpoint the location of gamma rays with an 8 percent certainty, important for astrophysics research, but they provide color information at a lower resolution. Together, the combined information from the CMOS/CZT array would produce spectacular images. (See table.)
“We configured the CMOS and CZT detectors and flew them in the Coronas-Photon [Complex Orbital Observations Near-Earth of Activity of the Sun] satellite,” Rao said. “These detectors are very robust and survived all the space qualification tests, such as shock absorption, vibration and thermal cycling.”
The bottom line for astrophysics imaging applications is that there are excellent alternatives to placing complex, expensive detectors aboard a research satellite. Many gamma-ray detectors use a thick direct-detection layer bonded to a CMOS readout chip and a single vertical connection per pixel. Direct detection offers large signals, energy discrimination and good absorption efficiency; however, such a two-layer “sandwich” detector is difficult and expensive to manufacture and has low resolution, and the total active area is typically a few square centimeters in size. Combining CMOS and CZT provides the best of both architectures at a lower price.

A coronal mass ejection, as viewed by the Solar Dynamics Observatory in June 2011. Courtesy of NASA/SDO.
A fated mission
The telescopes were launched into polar low-Earth orbit in 2009 and carried into space on the Russian solar research satellite Coronas-Photon. The overall mission objectives for the Coronas-Photon were to measure energetic particles produced by solar flares, the solar atmosphere and the relationship between solar activity and magnetic storms around Earth, but the mission ended after just nine months because of a power outage on the satellite.
The RT-2 was pointed at the sun to study the temporal variations in the energy spectra of hard electromagnetic radiation in the broadband energy range from 15 to 2000 keV; the fast timing of hard x-ray radiation in flares; the measuring of the linear polarization of hard x-rays in flares; the hard x-ray imaging of the sun; and the energy spectra, charge and mass composition of ions accelerated in flares.

The CMOS component of the RT-2 CMOS/CZT telescope is a RadEye1 CMOS detector from Rad-icon Imaging. Photo by Carter Dow Photography. Courtesy of Rad-icon Imaging.
All payloads aboard the satellite worked properly with good health parameters. Although no solar flares occurred while the satellite was in orbit, the RT-2 captured background images of gamma-ray radiation, enabling the researchers to calibrate and test their instrumentation. The variation in photon counts was recorded as the satellite passed through the South Atlantic Anomaly, North Pole and South Pole regions. Researchers continue to perform detailed analysis of the individual pixels generated within gray-scale images to understand the system completely. Plans are in the works to launch another satellite with some modifications to the imaging technologies.
The future of gamma-ray astronomy
Depending on whether telescopes are facing the sun or away from it, there can be huge temperature differentials for instrumentation on a satellite. One future research direction is to implement cooling chambers for temperature stabilization, which, in turn, would help control the dark current on instrumentation such as the RT-2. Gamma rays do not generate a lot of signal in the CMOS photodiode, so having low dark current and long integration times would create an optimized imaging environment.
Another future research direction is improving the signal-to-noise ratio. Rao theorizes that lower noise improves energy resolution, which would make it possible to do basic energy discrimination with CMOS detectors. Smaller pixel sizes could further lower the noise floor in the instrument, although there is a trade-off between pixel size, read noise and signal intensity.
Finally, the scintillator technology could stand to be more finely tuned for astrophysics imaging because the relatively high energy photons require good absorption efficiency. Scintillators are typically manufactured from rare-earth materials that convert gamma rays into visible photons. When the visible photons exit the scintillator, they are immediately absorbed by the photodiodes and, thus, generate an image. Researchers continue to search for the optimum scintillator material to absorb gamma rays: They look for cross section, which is the probability that a given material will absorb gamma rays and is proportional to the fourth power of the atomic number of the material.
CMOS imaging technology has offered a new dimension to data collection in gamma-ray research for its robust sensors with their high resolution and cost efficiency, Rao said. His approach of combining high-energy resolution and high-spatial-resolution sensors is creative and may well become a new standard for gamma-ray astronomy in the future.
Meet the author
Alana Achterkirchen is the director of business development and marketing for Rad-icon Imaging, a division of Teledyne Dalsa of Sunnyvale, Calif.; e-mail: [email protected].