Adrian F. Pegoraro, Albert Stolow, National Research Council Canada and Queen's University; Andrew Ridsdale, Douglas J. Moffatt, John Paul Pezacki, National Research Council Canada; and Yiwei Jia, Olympus America Inc.
Advanced optical imaging techniques can provide unprecedented details about the dynamics of life on a microscopic level. Such techniques typically rely on fluorescent molecules introduced into cells and tissue for labeling purposes; these probes may conceivably introduce artifacts or perturb the system under observation. Label-free imaging may be not only desirable but necessary in many applications, including certain in vivo and intravital imaging experiments.
Intracellular organelles are chemically distinct, being composed of different molecules. Coherent anti-Stokes Raman scattering (CARS) microscopy is a label-free, chemical-specific imaging modality based on the Raman spectrum, or vibrational fingerprint, of a molecule.1,2 CARS requires the input of two ultrashort laser pulses, called pump and Stokes (Figure 1), whose typically near-infrared frequencies avoid photochemical damage and allow for deep penetration into tissue.
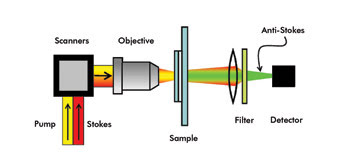
Figure 1. CARS microscopy provides label-free imaging.
CARS relies on the frequency difference between the pump and Stokes pulses being tuned to match a vibrational mode of the molecule of interest; the absolute frequencies of the two pulses are relatively unimportant. When this frequency difference matches the frequency of the specimen’s molecular vibration, a strong blueshifted anti-Stokes signal is generated, allowing molecule-specific imaging.
Successful CARS microscopy implementations, such as those based on the use of two synchronized picosecond laser pulses,2 rely on the fact that the pump and Stokes pulse linewidths match the spectral linewidth of the Raman band of interest. The CARS process offers many advantages over traditional Raman microscopy, primarily that its vastly stronger signal permits real-time imaging. The most successful application is arguably in imaging lipids, where the mode of interest is the CH2 vibration, an intense Raman mode having a broad spectral linewidth.
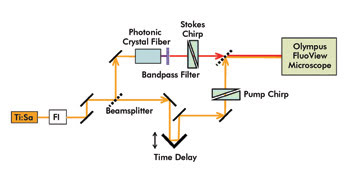
Figure 2. A simple CARS microscope. A beamsplitter splits pulses from an isolated femto-second Ti:sapphire laser. Half goes to a photonic crystal fiber to generate the Stokes pulses, followed by a bandpass filter, before being recombined on a dichroic mirror.
Multimodal imaging combines CARS with other optical techniques such as two-photon fluorescence (TPF), second-harmonic generation (SHG) or sum frequency generation (SFG); it is much more powerful than any single imaging modality. TPF and SHG signals, however, benefit greatly from the use of femtosecond, rather than the longer picosecond, laser pulses.
Because of their much broader bandwidths, it might be thought that femto-second laser pulses would present problems – i.e., poor spectral resolution – for CARS. However, although femtosecond pulses have broad bandwidths, well-known nonlinear optical methods exist for greatly enhancing resolution.
The simplest method, called “chirp,” involves stretching the pump and Stokes pulses equally so that they are longer in duration.4 By optimizing the degree of chirp in femtosecond pulses, we achieved a very simple yet high-performance multimodal CARS microscopy method that is robust, inexpensive and stable enough for imaging live cells – at 2 fps – over a period of days.3
To illustrate how femtosecond laser chirp can be used to optimize both sensitivity and resolution in CARS microscopy, imagine playing the piano with your left hand (denoting pump) and the right hand (Stokes). You set the distance between your hands, or frequency difference, to “probe” a given Raman resonance. To symbolize picosecond laser pulses, you carefully choose exactly two keys and, using one finger from each hand, play them simultaneously.
Using a single key for each hand reflects the idea of the narrow bandwidth of picosecond pulses. By contrast, femtosecond pulses can be 100 times broader – as with all the keys on the keyboard. Because in CARS microscopy it is only the frequency difference and not the absolute frequency that counts, you play all the keys on the keyboard in sequence – i.e., “stretch” the notes out – rather than at once; in other words, “chirping.” As long as you always keep your hands the same distance apart, you will keep a constant frequency difference and, therefore, a narrow Raman resonance. In this manner, you can use all the keys but maintain high spectral resolution.
Going a little further with this analogy, we can understand how the degree of chirping affects CARS spectral resolution. Chirping extensively is like playing all the keys of the piano with your hands a fixed distance apart – one pair of notes at a time. In this case, the spectral resolution is the same as if you only played two keys; i.e., picosecond pulses. Another option is playing with three or even five fingers from each hand, still keeping the hands equidistant – the same Raman resonance – playing all the keys in sequence. Clearly, spectral resolution is poorer – multiple fingers vs. one – but the advantage is that the sound is louder; i.e., bigger signals.
Raman spectra can be obtained simply and quickly by changing the time delay between the pump and Stokes pulses. Having user control over both the chirp and time delay of two femtosecond pulses allows for very simple, flexible and effective CARS microscopy.
Our optical arrangement3 incorporated a photonic crystal fiber to generate synchronized redshifted femtosecond Stokes pulses, permitting broad Raman tunability (~2500 to 4100 cm–1). The pump was sent to a variable time delay stage, permitting rapid scanning of the Raman spectrum. The chirp of the pump and Stokes pulses was controlled and varied using wedges of glass, typically adjusted to achieve nearly matched chirp rates. Typical laser powers before the Olympus FluoView scan head were 7 mW for the Stokes and ~50 mW for the pump. These powers were attenuated by about a factor of two upon passage though the system.
Imaging was performed using an Olympus FluoView confocal system with a 40× objective and condenser lens for forward collection – CARS and SHG. TPF signals were collected through the objective. Filters were used to discriminate the CARS, SHG and TPF signals from each other and the input pump and Stokes beams.
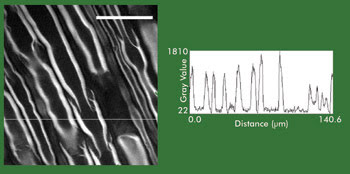
Figure 3. Forward-detected CARS imaging of fixed rat dorsal root nerves at 2850 cm–1 (lipid C-H stretch). The myelin sheath surrounds the neuronal axon and generates a strong CARS signal because of its high concentration of lipids. Scale bar = 50 μm; pixel dwell time = 8 μs. Reprinted with permission from Optics Express.3
Rat spinal nerves approximately 300 μm in diameter and consisting of bundles of roughly 100 myelinated axons, each about 15 μm in diameter, were imaged (Figure 3). The effective depth of field of the CARS signal was ~1.5 μm. The pixel intensity profile of the indicated line demonstrates the excellent contrast achieved. The spectral response of the myelin (not shown) proved that the signals observed were solely the result of resonant CARS and not changes in the nonresonant background.
An atherosclerotic lesion from a rabbit aorta was used as a test sample for multimodal imaging (Figure 4). The image is a projection of a 50-image data set recorded along the axial direction (1-μm interval between images). An extensive network of collagen surrounds the lipid-rich tissues. Importantly, all three signals are endogenous to this sample.
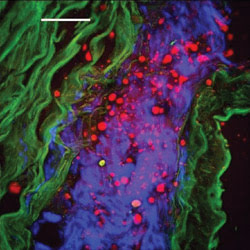
Figure 4. Label-free multimodal CARS microscopy of an atherosclerotic rabbit aorta. A 50-μm slice was imaged at 2850 cm–1 for lipids (CARS/red), collagen (second harmonic/blue) and smooth muscle elastin (autofluorescence/green). Scale bar = 50 μm; pixel dwell time = 8 μs. Reprinted with permission from Optics Express.3
We also performed imaging (movies) of lipid trafficking in live human liver cells over 48-plus hours, demonstrating that our optical arrangement is stable for long-term live-cell imaging.3
In conclusion, a simple, cost-effective, high-performance multimodal CARS microscope can be based on a single femtosecond laser. Practitioners of two-photon microscopy can add CARS microscopy without buying new lasers or giving up two-photon capabilities.
With chirp as a control parameter, microscopists can choose to optimize contrast in CARS imaging or enhance signals in various nonlinear optical processes in a multimodal microscope. Furthermore, by simply scanning the time delay between the pulses, they can achieve rapid multiplex CARS imaging without tuning any lasers.
Acknowledgments
We thank the Canadian Institutes of Health Research Regenerative Medicine and Nanomedicine team grant #RMF79032 for financial support; M. Sowa (NRC-IBD) and co-workers for the rabbit aorta sample; and NSERC, the Alberta Scholarship Program and NRC for funding support.
Meet the Authors
Adrian F. Pegoraro and Albert Stolow ([email protected]) are with the National Research Council of Canada in Ottawa and Queen’s University, in Kingston, Ontario; Andrew Ridsdale, Douglas J. Moffatt and John Paul Pezacki are with National Research Council Canada; and Yiwei Jia is with Olympus America Inc. in Center Valley, Pa. ([email protected])
References
1. J.-X. Cheng, X. Xie (2004). Journ Phys Chem B 108, p. 827.
2. J.-X. Cheng, A. Volkmer, L. Book, X. Xie (2001). Journ Phys Chem B 105, p. 1277.
3. A.F. Pegoraro, A. Ridsdale, D.J. Moffatt, Y. Jia, J.P. Pezacki, A. Stolow (2009). Optics Express 17, p. 2984 and references therein.
4. T. Hellerer, A.M. Enejder, A. Zumbusch (2004). Appl Phys Lett 85, pp. 25-27.