Dr. Jonathan S. Kane, irzoom.com
Although IR optics have
long been used for military, surveillance and industrial applications, it is only
recently that their full potential is being explored in biomedical settings. Common
applications are to study veins in vivo, to image certain cancers, and to sense
the absorption of oxygen in blood to determine the hemoglobin content.
Below, we explore a few examples of biomedical IR and discuss
how to specify or select an IR lens to maximize performance in your own application.
Four spectral regions are commonly used: near-IR (0.7 to 0.9 µm),
short-wave IR (0.9 to 1.7 µm), midwave IR (3 to 5 µm) and long-wave IR (8 to 12
µm). We refer to these as NIR, SWIR, MWIR and LWIR, respectively. The regions are
useful for sensing various features, offering a range of applications.
When added to the complement of analytical tools, the IR region
of the light spectrum allows for two additional determinants. The first is temperature
and the second, imaging within an expanded spectrum (beyond the visible).
Temperature and spectrum
Temperature historically has been used as a determinant of disease
or infection in individuals. In the mid- and long-wave IR, however, thermographic
scanning tools can offer abilities such as isolating individuals with elevated temperatures
in a crowd.
This technique offers broad applications for the control of infectious
disease, as demonstrated during recent SARS and H1N1 scares. In airports throughout
Asia, IR screening devices scanned for passengers with fever as they crossed security
checkpoints. Those with fevers were asked to step aside for further examination.
Such identification could minimize the spread of a contagion because those with
illness would be prevented from spreading it to other passengers and, ultimately,
other locations.
A given specimen often will transmit at one wavelength but absorb
in another. Sensing in IR allows us to see things that are not in the visible range,
and often to do so in a completely noninvasive way. Further, there are differences
within the four IR regions, so different information may be provided in one region
of the IR spectrum versus another.
A good example of how expanded spectrum can be used in biomedical
settings is the VeinViewer Vision imaging system from Christie Medical Holdings
Inc. of Memphis, Tenn. (Figure 1). This device emits near-IR radiation, which is
reflected back by the tissue surrounding a vein. No near-IR light is reflected back
from the blood inside the vessel, however, so the differential response between
vein and surrounding tissue is clear. A standard digital video camera captures the
reflected light, processes it electronically and projects it back onto the body.
The result? Hard-to-see veins can be observed clearly.
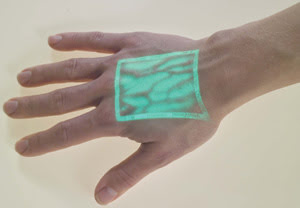
Courtesy of Christie Medical Holdings Inc.
Researchers at Cardiff University in the UK provide another example
of the opportunities presented by use of an extended spectrum. They have reported
using IR for optical “fly-throughs” of the eye, whereby full biopsies
can be performed in vivo without cutting into the living tissue.
Selecting an IR lens
Given the wide range of imaging opportunities between near-IR
and long-wave IR, several criteria should be considered when selecting an IR lens
for your application:
1. Wavelength - Because the absorption of materials is wavelength-dependent,
it is important to determine the range in which you need to image: NIR, SWIR, MWIR,
LWIR or a combination thereof. In addition, the optical materials change depending
upon the spectrum chosen for analysis, greatly affecting price.
2. Field of View - Do you need to see an entire hallway or just
a small portion of an eye? “Instantaneous field of view,” coupled with
the standoff distance, fully determines the effective focal length of the lens.
Typically, the smaller the field of view, the better the resolution.
3. Zoom Ratio - Many applications require several scales of resolution
within a single trial, making it highly desirable to have an IR lens that can zoom
in and out. For example, an area of the body may be examined to find an abnormal
lesion or hot zone, then magnified for more detail about the nature of the cells
in that area. Both continuous and stepped IR zoom lenses can be optimized for a
given application.
4. Expected Environment - Environmental concerns such as operating
temperature and space constraints will affect both the lens and camera system and
should be communicated clearly to the manufacturer or design team. Also important
is the desired standoff distance to the sample to be imaged. In public health applications
such as airport screenings, the standoff distance is relatively large as compared
with clinical settings, where the patient typically can come very close to the lens.
5. Camera Type - In the IR, the camera must be carefully matched
to the lens. Whether the camera is cooled or uncooled, the desired wavelength and
the available space (as it relates to camera size) are all important. In most cases,
this information can be found in an interface control document.
As in the visible, pixel size also is significant. In general,
the smaller the pixel size, the higher the resolution. The resolution of a 640 x
480 x 17-µm camera, used in a 1:1 imaging system, will be set at 17 µm, meaning
that each pixel is 17 µm wide.
6. The f/#-Diffraction Balancing Act - In the visible, diffraction
is relatively insignificant. This is not the case, however, in the IR. Understanding
the balancing act between f/# and resolution will save time and money when selecting
a camera and lens for your application.
Diffraction is discussed in terms of the blur circle, which can
be approximated using the following formula:
Blur circle = (2.44) x (Wavelength) x (f/# of the lens)
The f/# equals the effective focal length divided by the diameter,
so the lower the f/#, the bigger the lens.
From the standpoint of transmission in the IR, a lower f/# is
preferred. However, many biomedical applications require small lenses and sensors.
As the lens shrinks, the f/# goes up, light throughput drops, and the size of the
blur circle increases, limiting resolution.
On the contrary, if size is not an issue, the f/# of the lens
may be decreased, allowing more light through and enabling a higher resolution.
In these cases, resolution often is limited by the camera rather than the lens.
Consider the three examples below to see how changes to the f/#
of the lens affect system performance and how a good camera-lens match optimizes
performance. The black spots – also called Airy disks – shown in Figures
2A, 2B and 2C represent the blur circle, or physical diameter caused by diffractive
effects.
Figure 2A shows the lens set to f/1.5. In this example, lens performance
dominates the spot calculation and serves as the limiting factor. The Airy spot
does not play a significant role. In Figure 2B, the lens is set to f/6. Diffractive
effects dominate, and the lens geometric aberrations don’t play a part. In
this scenario, the system is limited by diffractive effects. If the pixel pitch
were 25 µm, the resolution would be at best two pixels.
Lastly, in Figure 2C, the lens is set to f/3, making the lens
and diffractive effects well-balanced. This would allow for optimal depth of field
and smallest size, while still passing as much resolution as possible by the physics
of the situation. Note that with the higher f/#, the aberrational control of the
lens is better because the light is not going to the maximum aperture. The spot
actually decreases in size.
Summary
Biological applications for the IR region of the spectrum will
continue to expand, driving the demand for smaller, more precise and range-specific
stock, and custom IR zoom lenses. We are excited by the new work in cancer research,
public health and other areas, which continue to push the boundaries of what can
be done and seen, using all ranges of the IR spectrum.
Meet the author
Dr. Jonathan S. Kane is president of Computer Optics Inc. and
division leader for the company’s IR division, IRZoom.com; e-mail: [email protected].