Biomedical lasers are experiencing strong growth, with a move toward diode and fiber lasers. Current trends in biomedical laser therapeutics are characterizing the dynamic nature of this important market.
MATTHIAS SCHULZE, COHERENT INC.
Lasers service a diverse range of biomedical applications, ranging from cosmetic fat removal and eyesight-saving photocoagulation to clinical blood cell counting. The major market driver for medical therapeutics has long been the aging “baby boomer” population of the Western regions of the world. The demands of this demographic have included medically necessary treatments, as well as aesthetic and other elective procedures.
But today, an increasingly affluent and appearance-conscious population in Asia, namely China, is making medical therapeutics a more significant market. Countries throughout Asia are working to bring their level of medical care closer to Western standards, as evidenced by the demand for instruments to support increased clinical lab testing. This has led to the development of a new generation of benchtop flow cytometers with an emphasis on lower cost and streamlined operation. This is, in turn, supported by compact, cost-effective optically pumped semiconductor lasers (OPSLs) and laser diodes. These benchtop instruments also target the increased point-of-care market in the West.
The increase in prosperity is also driving demand for elective procedures. Among them are numerous aesthetic treatments such as hair removal, body sculpting (i.e., lipolysis), skin resurfacing and teeth whitening. There is a similar increase in the demand in Asia for elective ophthalmic treatments as well, including LASIK and other vision correction procedures.
Diode-pumped fibers
The overarching technology trend in bioinstrumentation has long been a shift away from lamp-pumped solid-state and gas lasers to laser diodes and diode-pumped lasers (both OPSLs and fiber lasers). The reasons are the small size, high electrical efficiency and superior reliability of laser diodes compared to all earlier laser technologies used in biomedical applications. Moreover, laser diodes offer unmatched versatility in terms of output power and wavelength.
For therapeutic applications, typically there are one or more optimum wavelength bands that deliver the best selectivity — the laser produces a maximum effect while minimizing any unwanted collateral effects. By using different semiconductor compositions, the laser output wavelength can be set anywhere from the blue through the mid-infrared, with near-infrared devices generally offering the highest power levels. This wide choice of output wavelengths enables the laser system to be tailored to best match the needs of each specific application. These could include maximizing blood coagulation, tightening collagen, maximizing tissue ablation, maximizing penetration depth in soft tissue or limiting it to surface treatment, bursting target cells, killing dental pathogens and others.
In terms of output power, a typical individual diode laser emitter might produce at most a few watts (~5 to 15 W). However, a well-established modular architecture enables easy scaling to high powers. For example, numerous emitters can be fabricated on a single monolithic semiconductor array or bar with a total output of several tens of watts or higher. This is more than sufficient for most medical applications. The combination of multiple bars into a stack, or the use of multiple stack combinations by spatial, polarization or wavelength multiplexing, enables applications that require even higher power. Coherent’s DILAS also
offers a fourth format where several single emitters are efficiently combined in a single package (Figure 1).
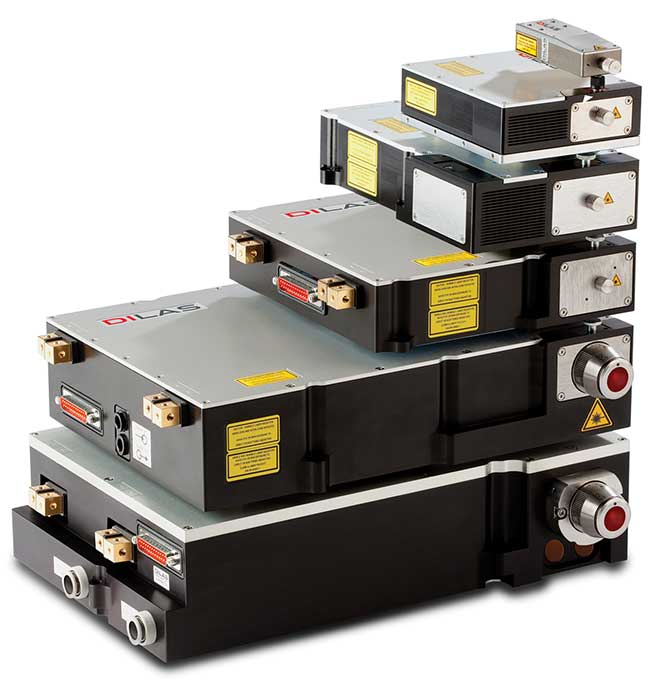
Figure 1. To support diverse therapeutic applications optimally, Coherent supplies diode lasers with a variety of scalable power levels and package types, including fiber coupling. Courtesy of Coherent Inc.
The flexibility and versatility of laser diodes power an incredibly diverse list of biomedical applications (Table 1). They completely dominate aesthetic applications where the largest single application is hair removal — this is serviced primarily by 760-nm laser diodes. This wavelength was selected to match the output of the alexandrite laser, which was formerly widely employed in this application. However, managing the trade-off between follicle and dermis damage becomes more difficult at this wavelength in darker-skinned patients because of the increased melanin in the skin. But by using shorter pulses (e.g., 30 ms or less) and longer wavelengths, hair removal is successful on darker skin types. Coherent provides diode lasers at both 808 nm and 1060 nm, as well as 760 nm, for this purpose.
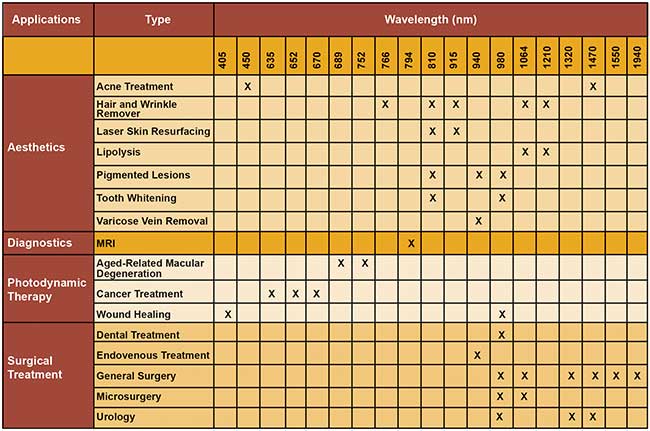
Table 1. A partial list of some of the biomedical applications for laser diodes. Courtesy of Coherent Inc.
Precision surgical applications
Most laser surgical procedures are based on selective tissue ablation. Until now, the majority of these procedures used either diode lasers at a wavelength of around 1 µm, Erbium:YAG lasers at 2.9 µm, or carbon dioxide (CO2) lasers with output around 10.6 µm; there were no readily available laser tools in the large gaps between 1 and 3 µm, and 3 and 10 µm. The absorption of laser light in human tissue is dominated by water absorption that varies quite dramatically in these wavelength regions, so they potentially represent unexploited surgical opportunities.
Recently, professor Thomas Milner and colleagues in the Biomedical Engineering Department at the University of Texas at Austin sought (via Coherent/Nufern) to build the optimum thulium (Tm3+)-doped fiber laser for potential development of an “image-guided smart laser knife” surgical system. The 1940-nm output of Tm3+ is close to the water absorption peak centered at 1900 nm. A typical target of Milner’s work is acoustic neuroma, a type of cancer found in the inner ear (Figure 2).
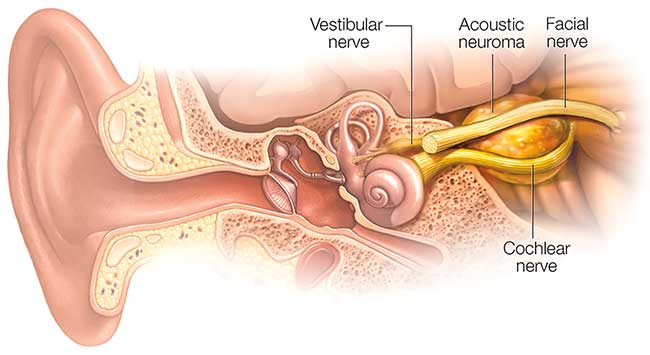
Figure 2. As a typical acoustic neuroma enlarges, it puts pressure on both the facial and cochlear nerves. It is usually a significant challenge to surgically remove the neuroma without damaging these nerves. Courtesy of the Mayo Foundation for Medical Education and Research.
Because of limited imaging capabilities combined with rough cutting and nonspecific cautery tools, the researchers explained that a conventional surgical approach to acoustic neuroma can result in damaged nerves and a distorted facial appearance. A Q-switched laser by Coherent has allowed highly localized ablation with minimized risk of damaging nearby vessels and nerves.
The carbon monoxide (CO) laser is a rare exception to the dominant trend toward solid-state solutions, as it produces a group of output wavelengths around 5 µm. Although they operate somewhat like the well-known CO2 laser, CO lasers had been largely restricted to laboratory applications because of the need to refresh the gas mixture and the requirement for cryogenic cooling.
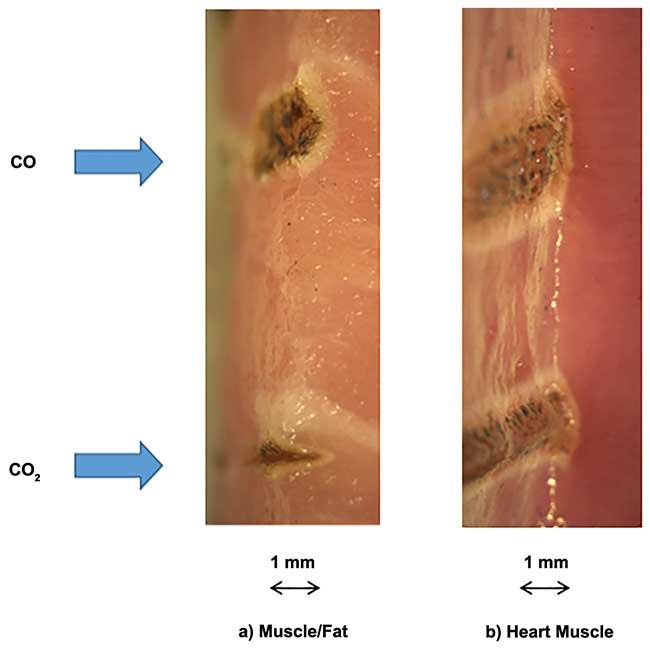
Figure 3. Comparison of porcine tissue samples cut with 8 W of CO and CO2 lasers at a speed of 4 mm/s (with the beam focused 2 mm below tissue surface) in fatty muscle (a) and heart muscle (b). The white zone shows the extent of coagulation. These cross-sectional microscope images were taken after perpendicular cuts with a scalpel. Courtesy of Art Photonics.
Applications scientists at Art Photonics in Berlin have been following up on earlier studies with experimental CO lasers by comparing them with a CO2 lasers of similar power. To date, the experiments have involved hydrated tissue samples in vitro. These indicate that the weaker absorption at 5 µm means that the laser power is absorbed over a large volume (compared to 10.6 µm), which results in a higher degree of photocoagulation compared to tissue ablation (Figure 3). Photocoagulation reduces bleeding, so these preliminary results indicate that the CO laser could be a superior tool for surgeries on highly vascularized organs and for vessel welding and similar precision surgical applications.
Excimer lasers
Excimer lasers are another important gas laser technology used in laser refractive surgical applications (LASIK). These procedures use the unique deep ultraviolet (193 nm) pulses from an ArF (argon fluoride) excimer laser to perform computer-controlled spatially selective ablation of corneal tissue. The deep UV wavelength is strongly absorbed with a penetration depth of a few microns. And the high photon energy serves to remove material by directly breaking the molecular bonds rather than by intense heating as with an infrared laser. Together with the short (a few nanoseconds) pulse duration, these factors enable precision micro-ablation of tissue with almost no peripheral thermal effects.
In the U.S., where elective procedures are more common than in other countries, laser refractive surgery is the single most popular elective surgical procedure. With a range of different procedures and variants now available, the key trends in vision correction surgery involve personalized treatments that provide subtle wavefront corrections for maximum patient benefit, as well as higher repetition rate lasers to reduce procedure times and minimize patient discomfort. In some of these applications, femtosecond ultrafast lasers have usurped the role of the mechanical cutting tool(s) for ancillary tasks that make up the complete procedure. But excimer lasers’ unmatched correction precision means they remain unchallenged as the best tool to perform corneal reshaping.
Clinical applications
In addition to therapeutic applications, laser-based instruments also play an important role in clinical laboratories and increasingly in medical facilities. Many of these instruments utilize laser excitation of fluorescent probes, mostly with visible laser wavelengths. Examples include cytometry, genetic sequencing, hematology, polymerase chain reaction (PCR), high-throughput drug screening and microarray scanners. Several trends, in both the West and Chinese markets, are driving a need for greater specificity, miniaturization and automation; this includes the development of benchtop, dedicated instruments configured for simplified and repetitive use in common tests, rather than the multifaceted flexibility of a typical flow cytometer.
These applications mainly use two complementary core technologies — laser diodes and OPSLs, in the milliwatts to watts range — in spite of advances in LEDs. Lasers can still deliver more useful photons in a more economical total package. Laser diodes are a more economical option and offer faster direct pulsing (up to 100 MHz), whereas OPSLs can deliver a superior beam quality that does not depend on output power. A comparison can be found in the August 2014 issue of BioPhotonics in an article titled “Streamlined Lasers Enable Lower Cost Instruments than LEDs.”
Here, the most interesting trend is in packaging: instrument builders increasingly looking to outsource photonic expertise to vertically integrated suppliers that can provide higher-level systems with guaranteed performance in the actual application. A few years ago, this involved laser diodes and OPSLs in an ultracompact package featuring an integrated controller. This enabled operation that was particularly useful in instruments with multiple wavelength options. It progressed to optomechanical modules, extending the plug-and-play concept further with fiber delivery.
More detail about this type of module is discussed in “Laser Combiner Enables Scanning Fluorescence Endoscopy,” featured in the November 2014 issue of BioPhotonics.
Over the last year, increased integration has yielded complete multiwavelength turnkey laser engines. These act as drop-in modules for several commercial instrument builders, where the positioning and focusing of the various wavelength beams are all independently controllable by the engineer assembling the instrument (Figure 4).
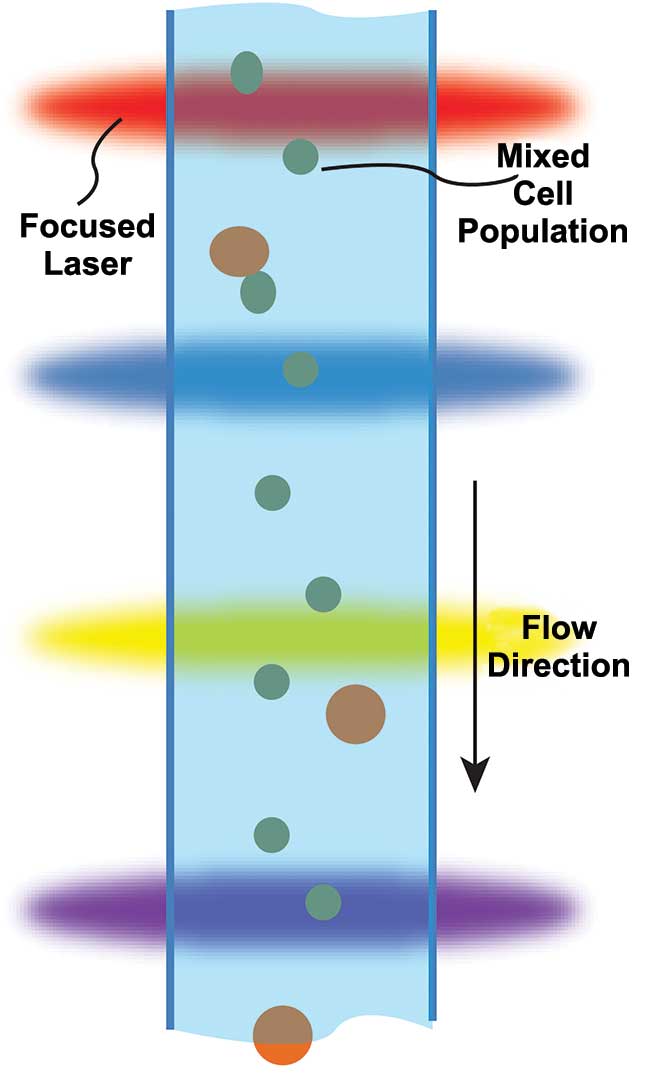
Figure 4. In flow cytometry, cells flow past multiple focused laser beams that are usually arranged as a sequence of elliptical foci. The use of multiple wavelengths enables cells to be assigned by a larger number of sort criteria. Courtesy of Coherent Inc.
The advantages of lasers in biomedical applications are much the same as in many other materials processing applications. Specifically, they include the ability to perform processes with high spatial and material specificity, and with minimal impact on surrounding material. For biomedical uses, in particular, this means an improved outcome and better quality of life for the patient.
Meet the author
Matthias Schulze, Ph.D., is the director of marketing for OEM components and instrumentation at Coherent Inc. in Santa Clara, Calif.; email: [email protected].