From alleviating the sensation of pain and treating depression to retrieving lost memories — or even implanting false memories — optogenetics applies precision science to the mysterious workings of our minds.
In a small laboratory with very little equipment, psychiatrist, neuroscientist and professor Karl Deisseroth began a high-risk project to render individual neurons photosensitive. Despite facing a great deal of skepticism from his peers, he remained convinced that if adjustments could be made to specific neurons, then scientists would finally have the tools to decode one of the most complex biological systems known to man — the human brain.
One year after he began, Deisseroth released his findings in a groundbreaking paper that presented optogenetics to the world. From quiet beginnings in his lab at Stanford University in 2005, the creator of optogenetics is today the much sought-after psychiatrist who is helping to improve the lives of patients who have nowhere else to turn.
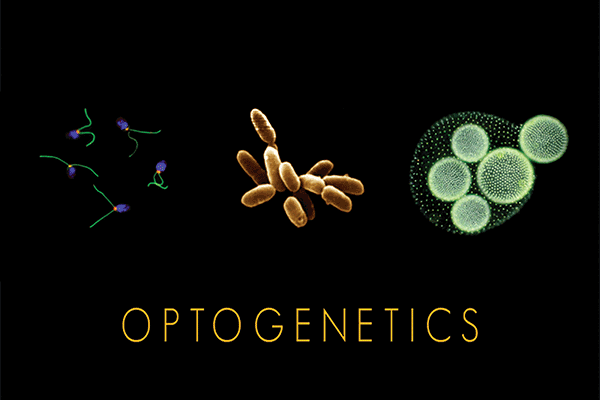
Some of the organisms from which optogenetic tools called ‘microbial opsins’ are taken. Courtesy of Feng Zhang and Karl Deisseroth.
Specializing in treating autism and severe depression, Deisseroth uses a combination of medications and electrical brain stimulation to assist patients for whom all other treatments have failed.
“The idea had been around in one form or another for many years but it was considered unlikely to work with microbial-based tools — the very same ones that, in fact, ended up working well, as we now know 10 years later, and so [it] did not receive much attention,” Deisseroth said. “I thought it would function to some extent but would require a lot of work, and indeed it took years for us to sort everything out to make it useful.”
With many years of challenging work behind him, Deisseroth’s efforts were recognized in 2010 when optogenetics was named Method of the Year by the journal Nature.

Illustration of how microbial opsins are first tested in a dish with glass microelectrodes and light delivery. Courtesy of Andre Berndt, Soo Lee and Karl Deisseroth.
“This was indeed rewarding — and timely — since it had taken us until 2010 to discover solutions to the key challenges involved, and there had been a lot of skepticism, well-founded and well-meant, but still skepticism, along the way,” he said.
Although it took about five years to catch on, optogenetics is now widely used around the world and has led to an explosion of published discoveries. Sleep and memory are two of the key areas under investigation, as well as understanding the mechanisms that underlie some of the most heartbreaking neurological disorders, including Alzheimer’s and Parkinson’s diseases.
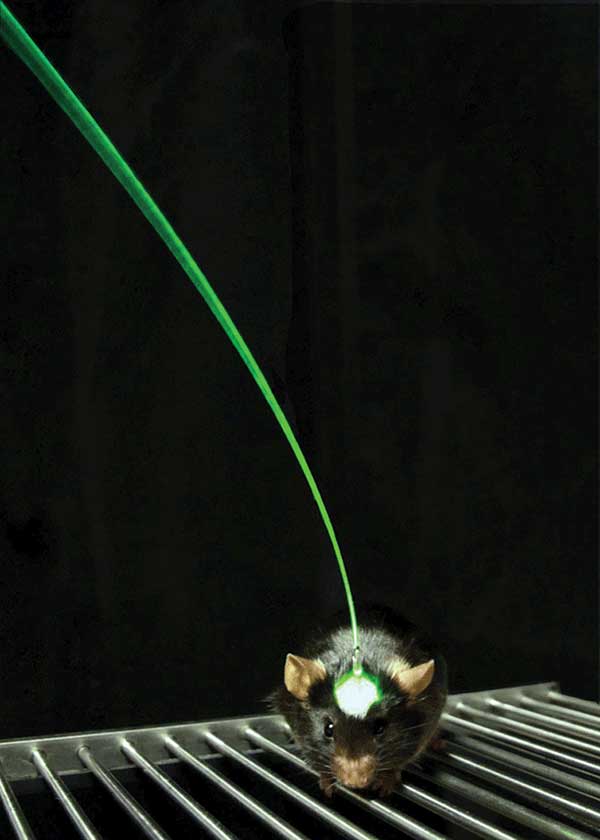
The fiber optic interface developed in the Deisseroth lab, and used in all of the studies described in this article, allows light delivery to deep brain structures during behavior. Courtesy of Inbal Goshen and Karl Deisseroth.
“Examples include defining how activity in brain circuits gives rise to complex behavioral states — relating to anxiety and depression, and identifying the cells and circuits involved in memory formation. These are fundamental questions in neuroscience,” said Deisseroth.
Tantalizing possibilities lie in the future of this unique branch of optics that crosses over into neuroscience. Linking a causal relationship to events or processes that occur in the brain with cognition or behavior expressed outwardly by living animals is only the first step. What could be revolutionary is to utilize optogenetics to manipulate an animal’s brain in order to alter its cognition and behaviors.
Inception: No longer science fiction?
Did you enjoy your dinner last night? When was the last time you felt really frightened? Retrieving such memories may require a few seconds of thought for us, but for neuroscientists at the RIKEN-MIT Center for Neural Circuit Genetics (CNCG) at the Picower Institute for Learning and Memory, at MIT in Cambridge, Mass., these few seconds are the subject of years of intense speculation.
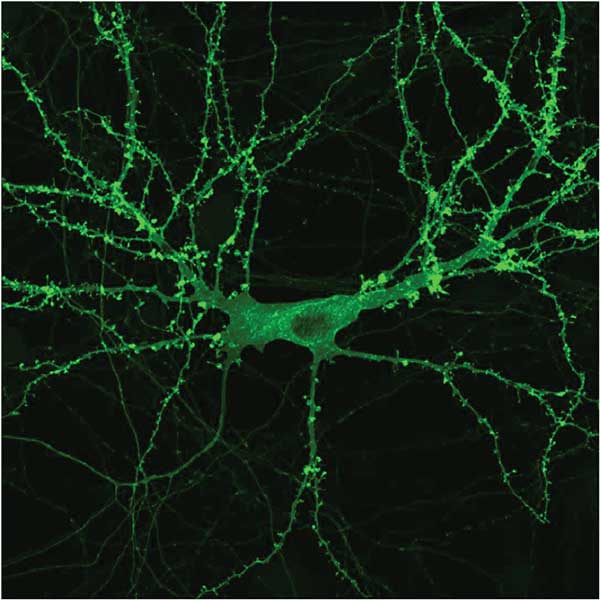
A neuron with a microbial opsin (coupled to a yellow fluorescent protein to visualize it) distributes throughout the cell. Courtesy of Priya Rajasethupathy, Charu Ramakrishnan and Karl Deisseroth.
“Neuroscientists have long thought that when humans or mice acquire memory of a particular experience, such as an enjoyable dinner last night, somewhere in the brain, one or more populations of neurons are activated by the experience, and enduring physical/chemical changes [that] occur in these neurons,” said Susumu Tonegawa, Picower professor in MIT’s department of biology and neuroscience, and director of RIKEN-MIT CNCG.
The changes are referred to as a “memory engram,” and the cells holding the changes are referred to as memory engram cells. For a subsequent recall of this memory, the engram cells have to be reactivated by recall cues.
The Nobel Prize-winning professor heads up a team at MIT who earlier this year revealed it was able to reactivate memories that could not otherwise be retrieved, using optogenetics.
Although the engram theory of memory has been around for at least a century, insufficient experimental technology prevented it from being validated. But in Tonegawa’s laboratory in 2012, the engram cells for context-specific fear memory were labeled with an opsin, and subsequently, blue light stimulation of these cells caused an animal to recall the memory in an entirely different context — one that should not have served as a natural recall cue.

CLARITY image. Courtesy of Kwanghun Chung and Karl Deisseroth.
“A subsequent study demonstrated that scientists can even implant — we call it ‘incept’ — false memory in the mouse brain,” added Tonegawa. “The challenge is how to extend the information obtained in animal models to human models. The invention of revolutionary noninvasive or low-invasive technologies will be needed, and God knows when that will happen.”
The optogeneticist’s toolkit
Unlike some of the conventional devices applied in neuroscience today, optogenetics enables a specificity and precision of causal testing, defining specific populations and projections in the brain, that would not be approachable by drugs and/or electrodes.
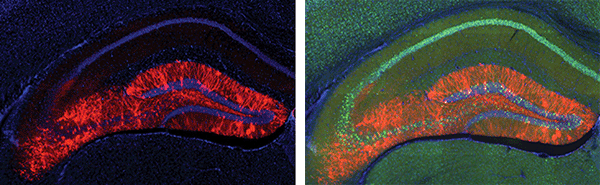
A cross-section of a positive memory. Seen here is the hippocampus; the brain cells glowing in red were previously active during the encoding of a positive memory and were also genetically tricked to respond to pulses of light. Optical reactivation of these cells elicits the recall of a positive memory. Ramirez et al., Nature (2015). Courtesy of Susumu Tonegawa/MIT.
As Michael Häusser, professor of neuroscience at the U.K.’s University College London, points out in his commentary on optogenetics in Nature Methods, published in 2014, light has numerous advantages for probing the functional properties of neural circuits in the intact brain. Not only is light noninvasive and precise, but it can also be used simultaneously at multiple wavelengths and locations.
Armed with a toolkit of proteins for activation and inactivation of neurons, lasers emitting different wavelengths and highly sensitive probes for imaging, today’s neuroscientists have never been better equipped to access the deep recesses of the brain.
Responding to the demands of its customers is the Canadian laser manufacturer Laserglow Technologies, based in Toronto, where developers have to keep pace with a field that’s exploding with research. But with greater exploration comes recognition of the limitations of current optogenetic approaches, which is in turn spurring further developments.

Engram synaptic plasticity. This image was very difficult to obtain and required high-resolution confocal imaging combined with 4-color immunofluorescence on single slices of hippo-campal tissue. This image beautifully represents the novel engram physiology data in Ryan et al., Science (2015). Importantly, this preparation shows individual engram and nonengram cells being studied simultaneously. Courtesy of Susumu Tonegawa/MIT.
“The growth of optogenetics was faster than any other known single neuroscience technique that we know of,” said N. Scott Browes, director of sales at Laserglow Technologies. “And the developments are coming as fast as papers can be published, or as fast as [a] researcher hit[s] the limits of current tools — opsins, reagents, light sources, etc. — which is when they demand improvements to various aspects of the tools for optogenetics.”
Even though LEDs have made some inroads to support this technique, it is the monochromaticity of lasers, as well as the relatively affordable nature of the systems and accompanying hardware to support spatial, amplitude and temporal control that is keeping lasers at the forefront of optogenetics research.
“Although light is what we are controlling, it is the electronics, the acoustics, the compact size and the overall robustness of the systems that often need improvements to provide a better solution,” Browes said. “For this we have developed, and are developing, new solutions to support researchers at the higher level of precision for input and control.”

Transparent mouse brain with CLARITY. Courtesy of Kwanghun Chung and Karl Deisseroth.
Barriers for working with some neural activity have been the response speeds and/or amplitude required to cause neuron stimulation or inhibition, as this relates to the intrinsic neuron frequencies that are above the speed of response for some of the early reagents/opsins.
For these areas, new tools are being developed and are now being realized. One such example is fast, low-light response media (such as cruxhalorhodopsin, a.k.a. “Jaws” by the Boyden lab at MIT), which enables research of the fast neuronal signals within auditory regions of the brain.
“Another important area is the homologation of knowledge using other imaging techniques such as MRI, and the many microscopy techniques — STED [stimulated-emission depletion], PALM [photoactivated localization microscopy], STORM [stochastic optical reconstruction microscopy], etc. — which, when coupled with optogenetics, reach new heights of specificity and results, often in real time,” Browes said.

A 473-nm diode-pumped solid state laser coupled to free space for use in wide-field channelrhodopsin stimulation. Courtesy of Laserglow Technologies.
In other modern advances, the use of two-photon illumination with spatial light modulator/holographic methods has enabled single-cell resolution optogenetic control in behaving mammals — an approach that was recently pioneered in Häusser’s lab in London.
Of mice and men
One of the biggest questions is whether some of the remarkable discoveries made so far in the small animal model will translate to the complexities of the human brain. The potential reach of using light to create a change in a human cell that can subsequently improve a problem is almost unlimited.
As a member of the advisory committee for the BRAIN Initiative, launched by the Obama administration in 2013, Deisseroth has been witness to a gradual acceptance of the technique.

A 473-nm blue beam is the primary channelrhodopsin wavelength, which, when used in free space, can be applied to microscopy applications (through the scope objective) in vitro. Courtesy of Laserglow Technologies.
“Once people get over that initial learning phase — it only takes a few days, the possibilities are nearly unlimited,” Deisseroth said. “Scientists vote with their feet and use what works. Although it took about five years to catch on — during which we were working on solving many of the key issues — optogenetics is now in use by thousands of laboratories around the world and has also led to thousands of published discoveries.”
A major challenge is detailed understanding of the wiring of cells controlled with optogenetics, which will be crucial for true reverse engineering of the brain.
Although today’s advances in optical techniques have opened the door to some exciting possibilities, it will take more than just optics to move forward along the path.
For example, modern tools for making brains transparent, such as the CLARITY technique which Deisseroth and his Stanford team devised in 2013, are valuable for investigating anatomical connections in fixed (dead) tissue from brains in which optogenetic experiments have been carried out. But the real need is for major computational advances that can cope with the very large imaging datasets produced.
“Neuroscience in general needs new ideas from statistics and computer science, as well as optics,” Deisseroth said.