Michael Feinberg and Paul Bierden, Boston Micromachines Corp.
Cutting-edge biological microscopy has enabled researchers to explore tissue at the subcellular level in vivo. Having the ability to observe physiological processes in vivo has led to breakthroughs in our understanding of cancer, eye disease and brain disorders and is paving the way for earlier diagnoses and more effective treatment.
Until recently, biological imaging devices have had resolution limitations that restrict the ability of researchers and clinicians to detect critical detail in vivo mainly because of tissue type, density and lighting. As light passes through tissue to reach the object of interest, the tissue induces wavefront aberrations in the light, causing images to be blurred and distorted and significantly reducing the contrast.
In vivo imaging also has struggled with a fundamental problem: using enough light to illuminate a sample while not using too much light, which can cause tissue damage. The use of adaptive optics has reduced the effects of optical distortion, providing clearer images down to the cellular level and minimizing potential damage to the tissue.
Fluorescence microscopy is a form of biological imaging that can benefit from improvements in adaptive optics, which may well become a fundamental component in this core instrument in biomedical research. When certain molecules are illuminated with high-energy light, they emit light of a lower frequency. This effect is known as fluorescence. Two-photon (also known as multiphoton) laser scanning microscopy (TPLSM) is a form of fluorescence microscopy that is being used in all major areas of biological research, from stem cells to cancer and heart studies.
TPLSM allows researchers to study changes in the physiological state of cells and tissues by combining laser scanning microscopy with long-wavelength multiphoton fluorescence excitation to enable deep, three-dimensional images for depths of up to 2 mm with submicron resolution obtained in vivo with minimal damage. With the technique, the imaging challenge is difficult because the images depend nonlinearly on laser power for excitation and, as researchers move farther into the tissue, because the fluorescent signals decrease rapidly.
Increasing the laser power partly compensates for the signal loss, but this adjustment is limited by the increased likelihood of phototoxicity to the sample. Adaptive optics solves this challenge by allowing research on thick, living tissue specimens that would not be possible using conventional imaging techniques.
Adaptive optics mature
Currently, the addition of adaptive optics aids TPLSM instruments in having consistent imaging capabilities, so that distortion is minimized and detail enhanced by helping to remove aberrations and misalignments present in the instrument as well as sample-induced aberrations. There are three main components of adaptive optics systems: a sensor that can measure wavefront aberrations, a wavefront corrector that can correct for the aberration, and a control system that takes measurements from the sensor and calculates the necessary movement of the wavefront corrector (Figure 1).
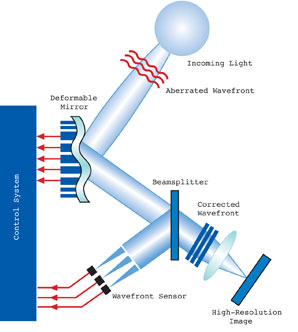
Figure 1. There are three main components of an adaptive optics system: a sensor to measure wavefront aberrations, a deformable mirror that can correct for the aberration, and a control system that takes measurements from the sensor and calculates the necessary movement of the mirror. Adapted from Professor Claire Max, director of the Center for Adaptive Optics, University of California, Santa Cruz.
A deformable mirror is the most commonly used wavefront corrector and the adaptive element of the adaptive optics system. Based on information provided by the controller, the mirror will change its shape to correct for the aberration in the wavefront, thus “cleaning up” the image. The deformable mirror is thin and flexible, with a number of control points behind it to adjust its shape. Microelectromechanical systems (MEMS) deformable mirrors are currently the most widely used technology in wavefront shaping applications because of their versatility and cost, the maturity of the technology and their ability to correct high-resolution wavefronts. Liquid-crystal spatial light modulators as well as macroscale deformable mirrors also have been used in this application.
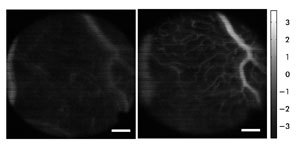
Figure 2. Mouse retinal imaging benefits from the addition of adaptive optics. Images taken without adaptive optics enabled (left) and with adaptive optics enabled (right) are shown. The scale bar represents –3 to 3 μm. (From David P. Biss, Daniel Sumorok, Stephen A. Burns, Robert H. Webb, Yaopeng Zhou, Thomas G. Bifano, Daniel Côté, Israel Veilleux, Parisa Zamiri and Charles P. Lin, “In vivo fluorescent imaging of the mouse retina using adaptive optics,” Optics Letters, Vol. 32, No. 6, March 15, 2007.)
Recently, the addition of adaptive optics to TPLSM has enabled scientists to see great detail in vivo of the anatomy of animals, shedding light on the exact makeup of biological structures. For example, Figure 2 illustrates in vivo fluorescence imaging of mouse retinal blood vessels before and after adding adaptive optics elements. As can be seen, the use of adaptive optics increases the resolution to the point that finer capillaries are visible; without its use, these capillaries are not visible. In addition, the use of adaptive optics increases the overall contrast in the image.
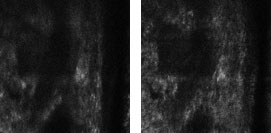
Figure 3. TPLSM images of mouse skull bone cavity show enhanced detail and contrast with adaptive optics (right). Bone imaging of mouse skull was taken at 180 m into the bone. (From Y. Zhou, T. Bifano and C. Lin, “Adaptive optics two-photon fluorescence microscopy,” Proc. SPIE, Vol. 6467, MEMS Adaptive Optics, Scot S. Olivier, Thomas G. Bifano and Joel A. Kubby, eds., January 2007.)
Another example of this enhanced capability is shown in Figure 3. A bone cavity in a mouse skull was imaged using TPLSM both with and without adaptive optics. Clearly, the bone cavity image acquired with adaptive optics shows improved intensity and resolution.
TPLSM and neuroscience
Recently, TPLSM has enabled groundbreaking advances in neuroscience that help researchers increase their understanding of brain disorders by studying neural dendritic spines and the relationship between blood flow and neuronal activity.
Dendritic spines are small protrusions or appendages that are present on neurons and are believed to help transmit and receive electronic signals from other neurons. Before the introduction of TPLSM, the basic physiological properties of dendritic spines could not be measured or observed because the volume of dendritic spines is 1 μm3 or less.
Researchers using TPLSM have learned that, by using time-lapse studies in the brains of living animals, spines can change significantly in shape, volume and number and can affect brain functions including motivation, learning and memory. Numerous brain disorders – such as autism, mental retardation and schizophrenia – are associated with abnormal dendritic spines.
Adaptive optics can play an important role in ensuring that the TPLSM instrument is always working at peak performance levels by correcting for misalignment in the optical system. This is critical during time-lapse studies and during research that depends on observing changes to tissue matter, particularly at the molecular level. Distortion can skew or influence results.
Discovery using TPLSM
New research using TPLSM from Northwestern University Feinberg School of Medicine in Chicago has revealed how schizophrenia works in the brain. Using a genetically engineered mouse model, investigators discovered that schizophrenia symptoms are triggered by a low level of kalirin in the frontal cortex of the brain, the part responsible for problem solving, planning and reasoning. Kalirin is a brain protein necessary for the development of dendritic spines.
With fewer dendritic spines, there are fewer neural pathways for information to travel, so the information becomes bottlenecked (Figure 4). This traffic jam results in hallucinations, memory loss and social withdrawal, the symptoms associated with schizophrenia (Cahill et al, 2009).
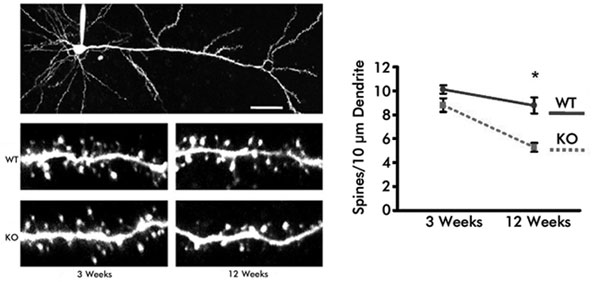
Figure 4. The images on the left show that, after 12 weeks, fewer dendritic spines are present in a genetically engineered kalirin knockout mouse (KO) as compared with an unaltered mouse (WT). This is found to be significant, as shown in the graph on the right. The reduction in spine density results in the symptoms associated with schizophrenia. Images are by Michelle Day using TPLSM. (From Michael E. Cahill et al, “Kalirin regulates cortical spine morphogenesis and disease-related behavioral phenotypes,” PNAS, Vol. 106, No. 31, pp. 13058-13063, Aug. 4, 2009.)
Michelle Day, a research assistant professor at Northwestern University in Chicago who acquired all TPLSM images for this research, said the instrument was found to be difficult to calibrate, requiring hours each month for alignment. With the addition of adaptive optics, calibration issues would be significantly reduced, increasing the availability of the instrument. By implementing adaptive optics, the need for expertise in calibrating the instrument on a regular basis would also be reduced, leading to lower cost of operation. Day believes that this could lead to more widespread use of the instrument because users such as biologists and practitioners of medicine would not need to be experts in the inner workings of the instrument to take advantage of the technology.
The examples listed can benefit from the use of adaptive optics. Introducing it into each of the optical setups renders the ability to capture images more dependable. Wider use of this technology could lead to new discoveries and advancement in the detection of disease and therapeutic treatment. TPLSM has become an important tool for learning more about brain function and its impact on disease. Adaptive optics greatly enhances TPLSM so that the instrument always works at peak performance levels and reduces optical distortion that could affect research results.
Discoveries such as those noted in this article are enabling researchers to make unprecedented medical research breakthroughs in diagnostics and therapeutic treatment for brain disorders and mental illness. With the use of adaptive optics, these discoveries no doubt will continue, leading to new discoveries as they have in other biological disciplines.
Meet the authors
Paul Bierden is president and CEO of Boston Micromachines Corp. Michael Feinberg is the company’s director of product marketing; e-mail: [email protected].