Fiber lasers have been employed in a variety of welding applications for many years. And, as laser technology further develops and diversifies, its uses in welding continue to expand.
WOLFRAM RATH, COHERENT INC.
Most traditional — i.e., nonlaser — welding techniques currently in use are variations of arc welding. In these methods, two pieces of metal are first brought into contact or close physical proximity. The edges of the pieces may have been shaped to facilitate their joining. A high voltage is launched between an electrode and the contact region, creating an arc that melts the material (or, in some cases an additional filler material, or the electrode itself). The melted material fills any gap between the workpieces — or overlays them — and then solidifies to join the parts.

Figure 1. Coherent/Rofin fiber laser oscillator schematic, including 12 diode laser pump modules, and 6 × 1 fiber coupling modules that inject pump light into the gain fiber and allow efficient extraction of the laser output. Courtesy of Coherent Inc.
The primary advantage of most arc welding methods is a relatively low cost, particularly in terms of the capital equipment expenditure. Furthermore, arc welding techniques are well-understood and widely employed, and standards for producing and testing them are well-established.
The major disadvantages of arc welding mostly derive from subjecting parts to high heat. This can result in microstructures in the melted material that yield poor strength in the weld joint and a relatively large heat-affected zone in the material adjacent to the weld. In addition, the penetration depth is low. While in recent years traditional arc welding technologies have been optimized to reduce heat input and deliver higher single pass penetration, laser welding is still the method of lowest heat input with the largest depth-to-width ratios. The parameters of the arc are influenced by the local electric field, and can therefore not be set independently. In contrast, laser welding offers fully independent parameter selection, and is the easiest technique to fully automate.
Laser keyhole welding
Most laser welding techniques can be classified into two basic categories: keyhole and conduction mode. Both methods are capable of autogenous performance — that is, with or without filler metal.
Keyhole — or deep penetration welding — is commonly encountered when welding thicker materials at high laser powers. With this technique, the laser is focused so as to achieve a very high power density at the workpiece. At the focus of the laser beam, the metal actually vaporizes, opening up a blind hole (the keyhole) within the molten metal pool. Vapor pressure holds back the surrounding molten metal and keeps this keyhole open during the process. The laser power is mainly absorbed at the vapor melt boundary and the keyhole walls. Both the focused laser beam and the keyhole continuously move along the welding path. At the front of the keyhole, new material is molten; at the back, it re-solidifies to become the welded joint.
The small size of the keyhole region results in a precise, narrow fusion zone, with a high aspect ratio (depth to width) as compared to arc welding methods. The highly localized application of heat means the bulk of the workpiece acts as an effective heat sink, allowing the weld region to heat up and cool down rapidly. This minimizes the size of the heat-affected zone, and reduces grain growth. Thus, the laser can generally produce stronger joints than arc welding, which is one of its primary benefits.
Laser welding also offers greater flexibility than arc welding, since it is compatible with an extremely broad range of materials, including carbon steel, high-strength steel, stainless steel, titanium, aluminum and precious metals. It can also be used to join dissimilar materials, as differences in material melting temperatures and heat conduction are of minor importance in the process.
In addition, laser welding delivers significant cost advantages over traditional methods, when all process steps are considered. In particular, the precise application of heat minimizes distortion in the weld and overall part, thus eliminating the need for post-processing in many cases. The ability to project the laser beam over relatively long distances with essentially no power loss makes it easy to integrate laser welding with other production processes, and lends itself well to integration with manufacturing robotics. Additionally, new product configurations with reduced flange sizes can be realized, which is critical for lightweight vehicles in the automotive industry.
Fiber lasers for welding
Fiber lasers match the requirements of keyhole welding extremely well. They typically offer output powers in the range of 500 W to 10 kW, and can readily achieve focused spot sizes in the necessary range between 40 and 800 µm, even at relatively large working distances. From a practical standpoint, the use of beam delivery fiber expands integration options and facilitates the use of the laser in the manufacturing environment. Finally, the high reliability, excellent uptime and favorable cost of ownership characteristics of fiber lasers make them an economically viable and attractive choice for production welding applications.
There are currently several manufacturers of high-power fiber lasers for welding and other materials processing applications. Figure 1 demonstrates the main elements of the fiber laser oscillator that is employed by Coherent/Rofin. The laser resonator is formed by a large mode area (LMA), Yb-doped, double-clad optical fiber and fiber Bragg gratings (FBG) for resonator mirrors. This is pumped from each end by a series of diode laser pump modules, whose outputs are fiber-coupled into the gain fiber.
Based on this design, one set of pumps and gain fiber can produce output powers of up to 3 kW. The output from up to four of these single-mode fiber laser modules can then be combined into one multimode fiber to achieve powers of up to 10 kW. Alternatively, the Coherent/Rofin standard cabinet supports splitting the output from a single fiber laser into four separate fibers through the use of the integrated fiber-to-fiber switches.
This modular construction approach makes it easy to provide a variety of options in terms of output power, delivery fiber diameter and beam parameter product. The benefit is the ability to readily adapt the laser beam characteristics to precisely match the exact requirements of a specific process.
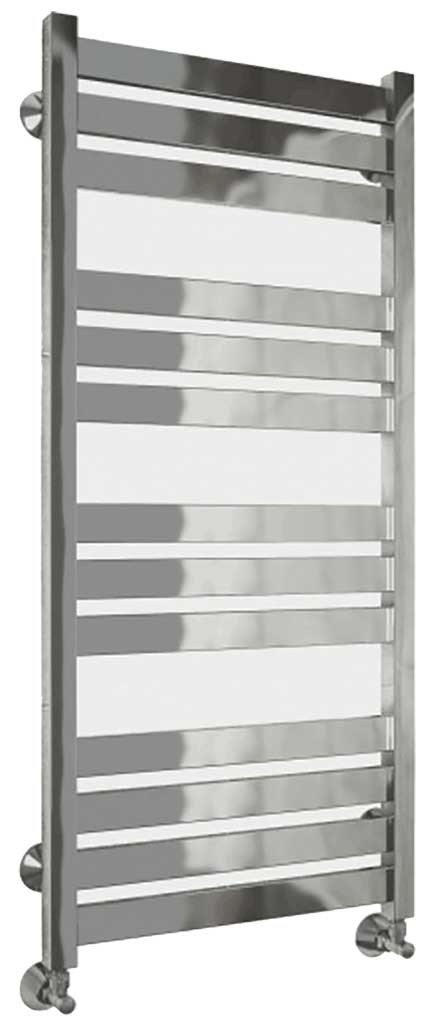
Figure 2. A towel heater, produced by a Russian manufacturer, uses an automated welding system from Rodomach Speciaalmachines BV. This system is based on a Coherent/Rofin fiber laser. Courtesy of Rodomach Speciaalmachines BV.
Some users of other fiber lasers have experienced laser damage or process inconsistencies caused by back reflections when processing highly reflective metals, such as copper and brass. Coherent/Rofin lasers utilize an optimized power generation and delivery technology, as well as sensors at different positions within the system, to protect laser components from such damage. These safeguards eliminate the problem of back reflections, and allow reliable welding of brass, aluminum and copper without any concern for damaging the laser.
Laser-welded towel radiator
Steam radiators for heating towels have become popular at gyms and spas worldwide. A Russian manufacturer of these towel heaters now employs an automated welding system based on a fiber laser that was developed by Dutch special purpose machine manufacturer Rodomach Speciaalmachines BV (Figure 2).
Previously, this Russian radiator manufacturer utilized the traditional tungsten inert gas (TIG) arc welding method by hand in its production. The goal of the radiator manufacturer was to transition all manufacturing to an automated system. Moreover, the process would need to accommodate a variety of different product configurations, including models with round pipes, as well as those having pipes with various other shapes. For all of these products, the desired welding depth is 60 percent of the pipe thickness, while the final assembly must withstand air pressures of 25 bars. Product appearance is also critical in this application; the manufacturer wanted to achieve a uniform, smooth seam weld (Figure 3), which is attractive and requires no post-processing. This is necessary because the final step in production is electro-polishing, which brings the stainless steel radiators to a mirror finish.
In order to develop a laser-based solution for this process, Coherent/Rofin ran trials for Rodomach at the Hamburg Applications Laboratory. These proved that the austenitic Cr-Ni-Steel AlSI 304 used by the radiator manufacturer was easy to laser weld. However, standard tooling could not ensure an optimum fit between parts for the entire operation, and as a result, a consistent high-quality seam could not be guaranteed.
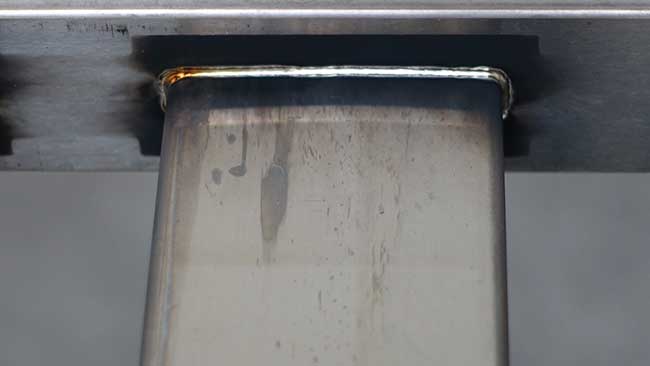
Figure 3.Detail of a weld seam produced by a fiber laser-based system. Courtesy of Coherent Inc.
Coherent/Rofin and Rodomach designed an approach that would clamp the part in a way that enables consistent welding, and also prevents part warping during the process. The particular solution was to replace the traditional, static clamp tooling used for welding with a servo-controlled clamping mechanism with integrated cooling and gas protection. With this clamping system, tube tolerances of the manifold and cross tubes are divided between two sides of the product. This method evenly clamps the part at all welding points, while the cooling dissipates the localized heat and prevents the joints from warping.
The testing also proved that a 2-kW fiber laser with a 300-µm delivery fiber, and focusing optics having a focal length of 300 mm, would be optimum for this application. This optical configuration provides a long depth of field, allowing the customer a high degree of process tolerance. The result is reduced scrap and improved productivity.
Rodomach configured the system so that through the use of a beam switch, a single fiber laser can feed two robotic welding stations that alternately process the two sides of the radiator (Figure 4).
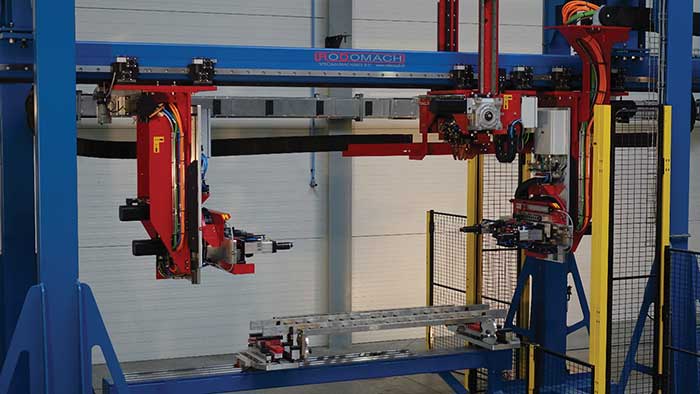
Figure 4. A system for automated welding of towel radiators. Courtesy of Coherent Inc.
“We pooled the control of the system, the two robots and the laser on to one terminal,” said Roel Doornebosch, managing director and owner of Rodomach. “This simplifies operation for the customer, who had expressed some concern at the outset that the system would be complex to operate due to their stringent quality requirements. The final system we delivered operates at a welding speed of 2 m/min, and provides welds that can withstand 250 bars of pressure — 10 times their original specification. In addition, the weld quality and cosmetics are consistently high.”
High-power fiber lasers have emerged over the past decade as an ideal tool for a wide range of welding applications. However, successfully deploying a fiber laser in a specific application requires more than just a high-quality source. In particular, partnering with a fiber laser company that offers support and expertise in process development and integration, together with a worldwide support infrastructure, is critical not only to bringing products to market, but also for long-term success.
Meet the author
Wolfram Rath is product manager of Laser Sources at Coherent Inc.; email: [email protected].