Jason Palidwar, Iridian Spectral Technologies
The future for photonics imaging is bright in a previously dark wavelength regime: The ability to image ‘invisible’ features such as thermal profiles, gases and other environmental constituents in a commercially viable manner has begun to open up new application areas for mid-wave and long-wave infrared sensor systems.
Over the past two decades, multilayer thin-film filters (TFFs) have become ubiquitous. These optical elements enable many varied photonics applications and markets in the visible (400-700 nm), near-infrared (700-1000 nm) and short-wave infrared (1000-3000 nm) wavelength ranges. Two primary application areas have been driven by – and have driven changes in – optical filters:
• Enabling wavelength division multiplexing, amplification gain flattening and dispersion compensation in high-capacity fiber optic telecommunications networks (1300-1600 nm).
• Providing more signal with less background to microscopists and spectroscopists investigating the presence and concentrations of specific chemical species through properties such as fluorescence or Raman scattering (400-1200 nm).
To address the development of these and other needs in the VIS/NIR/SWIR wavelength range, optical filter technology has seen an evolution in durability, filter shape complexity and cost. Filters no longer use soft coatings relying on lamination to protect them from the elements with an expectation of having to be replaced as they wear out and fade. Modern, energetically sputtered coatings provide filters engineered to meet the most stringent environmental and lifetime requirements. The development of in situ optical monitoring and automated coater control with dynamic design adjustment has enabled the successful production of optical filters with increasingly complex shapes, providing improved signal-to-noise ratios and facilitating multiband spectral performance. Improvements in coater uniformity and efficiencies of machine operation through load-lock systems have reduced filter manufacturing costs at the same time that the filters’ complexity has increased.
In parallel to this evolution in optical filter technology, market needs have arisen in spectral regions beyond VIS/NIR/SWIR. Once the domain only of the military and defense industries, which are less sensitive to cost, detectors employed in the mid- and long-wave IR (MWIR: 3-5 µm/LWIR: 8-12 µm) wavelength regimes have seen a substantial decrease in cost and an increase in regular commercial availability. This has given rise to commercial applications in markets such as surveillance, security, industrial process control, environmental monitoring and analysis, and medicine – which in turn has created a new set of requirements for optical filters.
Beyond thermal imaging
Working at longer wavelengths facilitates imaging and detection of lower-energy phenomena not otherwise observable in the visible and NIR ranges. Without the noise from background VIS-SWIR solar radiation, MWIR/LWIR systems can perform thermal imaging and thermography, detecting blackbody radiation emitted by organisms or machines. Hotter objects such as recently operated machinery or vehicle exhaust (100-500 °C) emit radiation at shorter MWIR wavelengths (3-5 µm); cooler, room-temperature objects (20-50 °C) such as humans or inactive machinery emit radiation detectable in the LWIR (10-12 µm), according to Planck’s radiation law1 (see Equation 1), where λ is the wavelength, T is the temperature, h is Planck’s constant, c is the speed of light, and k is Boltzmann’s constant.
Equation 1.

The typical applications of thermal imaging and thermography require standoff detection of radiation through atmosphere at a distance. Rayleigh scattering due to fog, mist, atmospheric or other gas molecules can inhibit this detection in the VIS/NIR/SWIR ranges, but for these small particles, this scattering is inversely exponentially dependent on the wavelength of the radiation to be detected (λ−4). As a consequence, imaging in the MWIR and LWIR wavelength ranges can see through dust, fog, clouds and mist. To selectively analyze specific thermal ranges, optical bandpass filters with specific center wavelengths can be employed in these camera systems via a filter wheel to “see” only in the region of interest while blocking out undesired background signals.

Figure 1. A nondispersive IR gas sensor is a simple device comprising an IR light source, a gas sample volume and an IR detector.
There are, however, specific molecular absorption bands with the MWIR/LWIR that inhibit transmission. Water exhibits a strong absorption band between 6 and 8 µm, creating an atmospheric “dead zone” between the MWIR and LWIR. This broad water absorption band limits the useful range for thermal imaging and thermography, but the narrow absorption peaks associated with other atmospheric and industrial gases have made gas detection and analysis a strong growth application in the rest of the MWIR/LWIR.
Two specific detection technologies requiring unique optical filter characteristics have emerged: nondispersive IR (NDIR) gas detection and remote sensing employing multispectral imaging (MSI).
NDIR gas detection
The ability to detect the presence and concentrations of specific gas molecules has value in many applications and markets. Gas detection and sensing are useful in many applications including industrial process monitoring of CH4, CO2 and CO, where the presence of a specific gas may indicate a key process parameter such as combustion or food spoilage; automotive exhaust or cabin air quality (NOx and CO2); monitoring of building air quality and safety (formaldehyde and CO); medical and health gas monitoring in anesthesiology, sleep apnea and Breathalyzers; flame detection; and motion sensing for crowd populations.
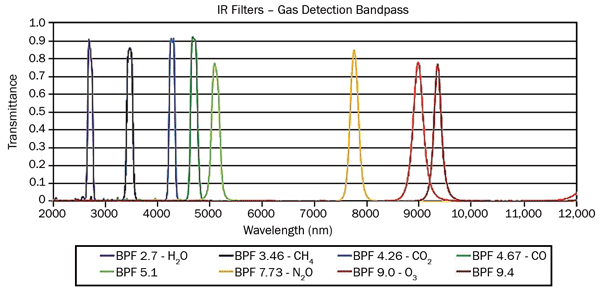
Figure 2. Gas detection bandpass filter curves. New durable sputtered materials allow coatings to be designed and deposited with narrow passbands, offering high selectivity for detection of specific gas absorption lines.
IR gas sensors provide an optical approach to detecting the presence and concentration of these specific gas molecules. Unlike electrochemical sensors, IR sensor systems do not rely on chemical reactions, so their longevity is higher; they do not suffer from performance degradation over time.
NDIR sensors are relatively simple devices consisting of an IR light source, a gas sample volume and an IR detector.2 Key to their functionality is the presence of a wavelength-selective optical filter situated in front of the detector (Figure 1).
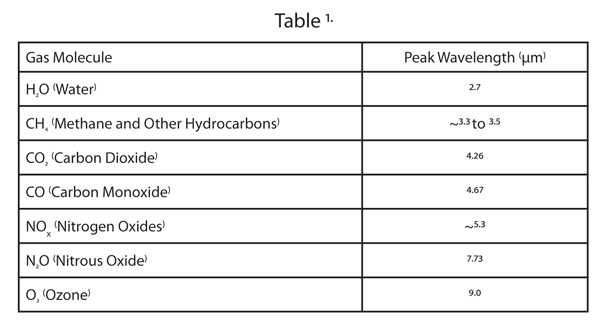
IR light propagating to the detector will be absorbed according the unique spectral fingerprint associated with the species of gas molecule present (see Table 1). If an optical filter’s bandpass region corresponds to the peak absorption band of a selected gas (or gases), then the intensity of filtered IR light reaching the detector will depend only on the concentration of the chosen gas. If none of the chosen gas is present, the intensity will match a reference level. In the presence of the selected gas, the intensity at the detector will vary with inverse proportionality to the concentration of gas according to Lambert-Beer’s law (see Equation 2), where I is the intensity of light at the detector, I0 is the reference intensity (no gas present), k is the absorption coefficient constant for the system, and P is the concentration of the gas being measured.
Equation 2.

The optical filters in these NDIR sensor systems are analogous to narrow-emission bandpass filters used for fluorescence detection and analysis in the visible wavelength range. However, the metal-oxide dielectric coating materials and glass substrates commonly used in the VIS range are not transparent in the MWIR/LWIR. Suitably transparent substrate choices for the MWIR/LWIR include Si, Ge, sapphire and ZnSe, to name a few, but, historically, coating materials for these regions often could only be deposited by evaporation techniques, making them less robust. Recently developed sputtering methods that can deposit IR-transparent materials have enabled the production of more robust optical filters. These new durable sputtered materials allow coatings to be designed and deposited with narrow passbands (<5 percent of the center wavelength), high peak transmission (>70 percent) and broad blocking (UV-LWIR), providing the high selectivity needed for detection of specific gas absorption lines.
Traditionally, IR gas sensor systems have been more costly than electrochemical systems. However, with the increasing availability of low-cost MWIR sensors and improvements in MWIR “gas bandpass” optical filters – reduced cost; improved availability; and greater selectivity, durability and affordability – the demands for NDIR gas sensors are expected to continue to experience significant future growth. New demands for these gas sensors will continue to emerge as sensors proliferate in all aspects of society.
Remote sensing and MSI
MWIR/LWIR detectors have become more affordable, but some applications still require optimization of the detectors and systems to analyze multiple different phenomena and constituents in a single device. In particular, Earth-observation instrumentation deployed on satellites for remote sensing of environmental characteristics must meet budgets for size, space and weight. Also, the ability to “fuse” images of different time-varying phenomena is very important to demonstrate relationships and dependencies between these phenomena. By coupling optical filters with spatially varying spectral performance (multizone filters) with a camera/detector, optical engineers have created MSI systems, minimizing cost and payload size, and providing “snapshot in time” image fusion of multiple analytes.
MSI systems in the VIS-NIR can be constructed using photolithography processes to pattern the different spectral zones on a monolithic substrate. For the MWIR/LWIR, challenges to this traditional manufacturing approach have included coating thicknesses as well as different coating and substrate materials – new technology that assembles arrays from individual MWIR/LWIR bandpass filters solves this problem. Additionally, new deposition processes and materials create robust, spectrally selective individual filter elements (analogous to the gas bandpass filters described above). Extensive experimentation has led to assembly techniques that build arrays that achieve minimal pixel loss through well-controlled optical zone sizes and minimal transition zones, while meeting the requirements for the durability and reliability needed for instruments destined for space (radiation insensitivity, structural integrity, etc.).
Figure 3 shows a recent example of this technology’s application. Developed by Iridian Spectral Technologies under subcontract from ABB Canada for the Space Technology Development Program of the Canadian Space Agency, this optic consists of 10 different spectral bands in the MWIR/LWIR, all in a footprint the size of a penny.
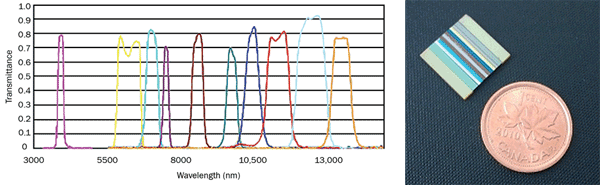
Figure 3. Spectral curves (a) for the compact MWIR/LWIR filter array (b) developed under subcontract from ABB Canada for the Space Technology Development Program of the Canadian Space Agency.
The different spectral bands in this optic allow for simultaneous imaging of cloud height, ozone levels, atmospheric ash, wind and humidity levels, fog, fires, ice and more.3 When this technology goes into orbit in 2017, it will present new capabilities to measure and monitor these environmental characteristics in the Canadian arctic, improving weather forecasting and climatology.
Other remote-sensing applications could be facilitated by leveraging MWIR/LWIR multizone filters, or by combining these with VIS-SWIR filters to provide an even larger spectral coverage. These applications include agriculture, forestry, and tracking of the effects of natural or man-made disasters (volcanic ash clouds, forest fires).
Meet the author
Jason Palidwar is an account manager at Iridian Spectral Technologies in Ottawa, Ontario, Canada; email: [email protected].
References
1. A. Rogalski and K. Chrzanowski (2002). Infrared devices and techniques. Opto-Electronics Rev, Vol. 10, No. 2, pp. 111-136.
2. International Light Technologies. NDIR Gas Sensor Lamps – Technical Application Information. http://www.intl-lighttech.com/applications/light-sources/ndir-gas-sensor-lamps.
3. A.P. Trishchenko and L. Garand (Feb. 7, 2011). Canadian Polar Communication and Weather (PCW) satellite system: New capabilities for mapping Artic snow and ice dynamics from Highly Elliptical Orbit. http://www.asc-csa.gc.ca/pdf/PCW_trishchenko_garand_2011-02-07.pdf.