A photonic crystal fiber infiltrated with liquid crystal displays all-optical modulation.
Thomas Tanggaard Alkeskjold, Technical University of Denmark
Photonic crystal fibers, usually made from silica, have a microstructured cross section of airholes running along their length. In recent years, much attention has been focused on the development and design of the fibers for various applications, including supercontinuum generation, nonlinear signal processing in high-speed optical time domain multiplexing communications systems and high-power double-clad fiber lasers.
Such applications use fibers with static properties. In others, such as those involving dispersion, polarization or attenuation, however, the transmission properties of the fiber should be dynamically tuned and trimmed, while keeping the signal within the fiber.
Photonic crystal fibers also are candidates in these cases, because the airholes provide access to the guided light in the core and because liquids can be infiltrated into the capillaries.1 The optical properties of the liquids usually are easier to modify than the silica’s. This approach, therefore, paved the way to components based on tunable photonic crystal fibers.
These fibers guide light using two different principles. The first is modified total internal reflection, which relies on guiding in a high-index core surrounded by a low-index cladding material. The second involves guiding in a low-index core surrounded by a cladding arrangement of high-index rods. Light coupled into the core can be guided through antiresonant reflection from the rods, which form a sort of two-dimensional Fabry-Perot cavity surrounding the core.2
If the rods are periodic, they form a photonic bandgap effect; when calculating the allowed optical modes in the periodic structure, gaps appear between bands of allowed modes. A guided mode can exist within these gaps when a low-index defect is created in the periodic structure. The periodicity is not a restriction on guidance, but it does reduce the transmission loss considerably.
The photonic crystal fiber guides light by the principle of modified total internal reflection when the holes are filled with air or with a material with a lower refractive index than that of silica. Infiltrating the airholes with a high-refractive-index oil or liquid crystal transforms the fiber into a photonic bandgap guiding type, which guides light in the low-index silica core.3,4
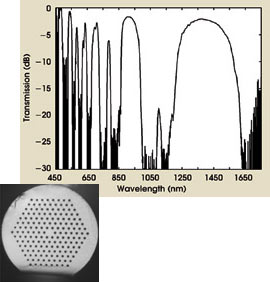
Figure 1. The graph shows the transmission spectrum of a photonic crystal fiber infiltrated with a nematic liquid crystal. The inset is an optical micrograph of the fiber used in the experiment. ©Optical Society of America.
To a large extent, the optical modes of a single liquid-crystal-filled hole determine the spectral properties of a photonic crystal fiber infiltrated with a nematic liquid crystal (Figure 1). Because liquid crystals are highly anisotropic, the optical modes are determined not only by the refractive indices of the liquid crystal, but also by the alignment within the hole. Therefore, changing the alignment and/or the refractive indices of the liquid crystal can modify the spectral properties of the fiber.
Nematic liquid crystals are known for exhibiting very large thermo-optic effects as a result of the existence of crystalline and isotropic liquid phases, which depend on temperature. When the liquid crystal is doped with a small amount of an absorbing dye, this high thermo-optic coefficient can be exploited optically to modify its refractive index and, thereby, to tune the spectral properties of the liquid crystal photonic bandgap fiber by optical means.5
In an experimental investigation of this effect, a 532-nm modulated pump laser and a 1620-nm CW probe laser were coupled into the liquid crystal photonic bandgap fiber, which was doped to absorb at the pump wavelength (Figure 2, bottom). The temperature of the liquid crystal was controlled externally so that the pump wavelength was located outside a bandgap to increase the coupling to the cladding modes, creating an overlap between the pump and the liquid crystal.
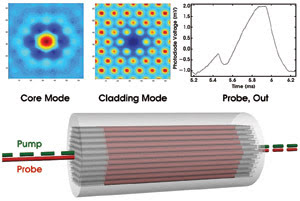
Figure 2. The dye absorbs the pump light, raising the temperature and shifting the bandgaps toward longer wavelengths. The probe radiation emerges with the same modulation as the pump light. The graph shows the probe’s response to a 500-μs square-wave modulated pump pulse.
The probe wavelength was located on the edge of a bandgap so that the laser beam was amplitude-modulated as the bandgap shifted under heating by absorption of the pump light in the dye. As a result, the 1620-nm beam emerged from the fiber with the same modulation as the 532-nm pulsed light. Effectively, the input signal was optically converted from 532 to 1620 nm.
When pump pulses with the same timescale as the thermal diffusion time of the liquid crystal rod (approximately 100 μs) and a peak pump power of only 2 to 3 mW were applied, a thermal response time of 100 μs was observed (Figure 2, upper right). It is estimated that the response time could be reduced by an order of magnitude by optimizing the structural parameters of the photonic crystal fiber.
References
1. B.J. Eggleton et al (Dec. 17, 2001). Microstructured optical fiber devices. Opt. Express, pp. 698-713.
2. N.M. Litchinitser et al (April 19, 2004). Application of an ARROW model for designing tunable photonic devices. Opt. Express, pp. 1540-1550.
3. R.T. Bise et al (March 17, 2002). Tunable photonic band gap fiber. Proc. Optical Fiber Communication Conference and Exhibit, pp. 466-468.
4. T.T. Larsen et al (Oct. 6, 2003). Optical devices based on liquid crystal photonic bandgap fibres. Opt. Express, pp. 2589-2596.
5. T.T. Alkeskjold et al (Nov. 29, 2004). All-optical modulation in dye-doped nematic liquid crystal photonic bandgap fibers. Opt. Express, pp. 5857-5871.
Meet the author
Thomas Tanggaard Alkeskjold is a doctoral student at Technical University of Denmark in Lyngby; e-mail: [email protected].