Latest technological developments create interesting trade-offs for clinicians and researchers.
NISHANT MOHAN, WASATCH PHOTONICS
Optical coherence tomography (OCT) is among the most widely used in vivo optical diagnostic techniques. This high-resolution 3D imaging modality, with market size approaching $1 billion, has established itself as an indispensable tool for ophthalmology and is seeing growing acceptance in interventional cardiology, dermatology and nondestructive testing.
OCT combines micron-level resolution with high speed and penetration up to 2 to 3 mm in the scattering tissue. In addition, instrumentation is relatively inexpensive and portable when compared to other 3D medical imaging modalities, such as MRI and CT.
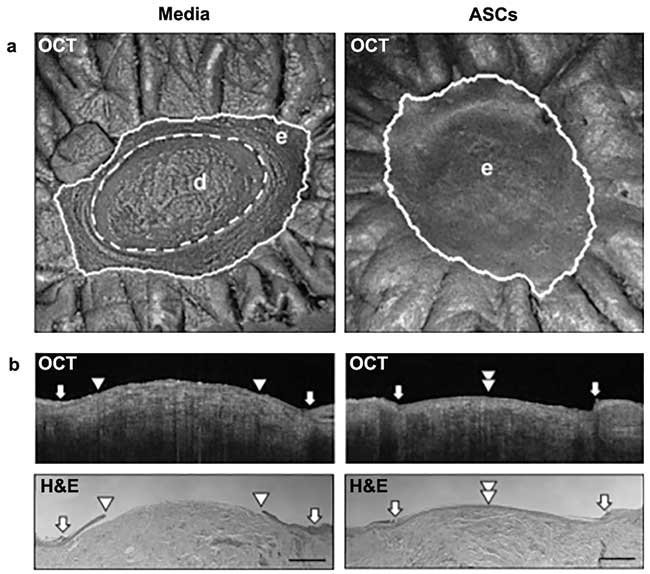
Figure 1. An example of using SS-OCT for studying therapeutic potential of allogeneic human adipose-derived stem cells (ASC) in ex vivo wound models. En face (a) and cross-section OCT (b). Comparing the two sides, one can clearly see reformation of epithelial layer when the ASC treatment is applied (see reference 2). Courtesy of Irena Paster, University of Miami.
Currently, nearly one OCT eye scan is performed every second, helping patients with conditions such as glaucoma, age-related macular degeneration (AMD) and diabetic retinopathy. Use of OCT for just one disease scenario of neovascular AMD has saved the U.S. government $9 billion in excessive medical costs1. From these savings alone, the return on investment is more than twentyfold for all of the investment made by the U.S. government in OCT technology.
At its core, OCT is a low-coherence interferometric technique. The earlier versions used time-domain interferometry. However, the technique really came into its own when Fourier-domain versions of OCT were introduced. In this implementation, interference of different wavelengths or colors illuminating the sample are recorded separately, and a depth profile is obtained using Fourier transformation to convert the data from wavelength domain to image domain.
(For details on OCT technique, see www.wasatchphotonics.com/oct-tutorial.)
Two main variations of Fourier-domain OCT have been developed over the last 15 years: spectral-domain OCT (SD-OCT) and swept-source OCT (SS-OCT). The difference in these two modalities comes from the mechanism employed to measure interference corresponding to different frequencies. SD-OCT shines different frequencies of light simultaneously on the sample and then spatially separates the frequencies using a spectrometer. SS-OCT uses a laser that “sweeps” through different frequencies over time to record interference as a function of frequency or color.
Both of these techniques rely on the same physical principle for image creation, but the implementation details of a swept laser and a spectrometer create interesting trade-offs. These techniques are now widely used, and there is a healthy competition in technology development to prove the dominance of one over the other. This has given interesting options to developers and end users, and it has called for due consideration in choosing the right modality for a specific application.
SS-OCT employs a class of lasers that changes its very narrow frequency line swiftly over time, which has given it two differentiating characteristics over traditional SD-OCT: high speed and deeper imaging.
SS-OCT is the technique of choice for several specialized applications, particularly in cardiovascular imaging and dermatology. Both of these applications require deeper imaging.
In intravascular OCT applications, the imaging catheter is situated in the lumen, and the area of interest includes both the lumen space and the scattering tissue. This makes long imaging range particularly important. In addition, detection of potentially vulnerable plaque is a critical piece of information obtained via OCT, which is possible only if the light can penetrate and image a few millimeters deep in the tissue.
Dermatology applications, including detection, monitoring and treatment of skin cancer and the wound-healing process, similarly require penetration through very scattering tissue medium. Recently, SS-OCT has been used to study therapeutic potential of allogeneic human adipose-derived stem cells (ASC)2 (Figure 1). As an in vivo technique, OCT allows a time sequence study and can be an invaluable resource for development of similar therapies.
The second key advantage of SS-OCT has been higher speed. In general, processes that determine the speed of these systems, such as an element based on oscillation of MEMS or on delay and dispersion through optical components, can achieve much faster speeds than can processes based on the transfer of photoelectrons, as in the case of SD-OCT. High speeds are critical in intravascular imaging, where blood has to be flushed from the artery momentarily for the imaging to be performed.
In addition, SS-OCT is often used in catheter-based imaging instruments. The rotation of imaging fiber can result in imaging artifacts because of polarization changes and is compensated for by using dual detectors. The detectors are less complex in SS-OCT, making it easier to employ dual detectors with this modality.
Recently, SS-OCT has seen growing acceptance in ophthalmology. Multiple SS-OCT ophthalmic instruments, including PLEX Elite 9000 from Carl Zeiss Meditec and DRI OCT Triton from Topcon Corp., have been introduced.
Here, as elsewhere, SS-OCT brings the advantage of high speed, which allows a wider field of imaging. In addition, deeper imaging provides a better view of choroidal structures below the highly scattering retinal epithelial layer. This makes SS-OCT suitable for imaging important disease markers such as choroidal neovascularization. Some of the advantages of deeper imaging in commercial swept-source instruments come from the choice of wavelength at 1050 nm and state-of-the-art optical designs and are not the result of fundamental difference between the spectral-domain and swept-source modalities.
SD-OCT was the first Fourier-domain variant to be commercialized. It quickly gained popularity in ophthalmology because of several advantages over existing time-domain OCT. SD-OCT, still the most widely used variant, has the advantage of providing higher axial resolution than SS-OCT. This comes from the simplicity of the light source, which provides more flexibility in the choice of operating wavelength and bandwidth. Higher resolution is critical in retinal imaging to easily resolve different layers. Ability to choose shorter wavelengths also provides better contrast for the inner retinal structures, such as ganglion cells. Spectral-domain also has been extended to perform visible-light OCT, which can provide critical biological parameters such as blood oxygenation levels.
Another differentiating feature of SD-OCT is its low cost. This is primarily the result of the use of components that are developed in larger volumes and manufacturing techniques that have been refined for developments related to other applications.
There is a constant pressure to reduce costs in health care, which then trickles down to reducing costs of the medical devices. This has been a driving factor behind the continued success of SD-OCT in ophthalmology. In addition, the growing applications of OCT for nondestructive testing and material inspection are inherently cost-sensitive. Spectral-domain becomes a natural choice in such cases. However, costs are driven by several complex factors, and users should carefully evaluate the cost structure for their specific applications.
In general, SD-OCT has been the modality of choice when high resolution and lower cost are key factors, while SS-OCT has grown in popularity over the past few years, particularly when faster and deeper imaging is required.
However, recent developments in SD-OCT has put it back on the map for applications that require high speeds
and deeper penetration in the tissue while maintaining high resolution and lower costs. These developments are closely associated with advancements in OCT spectrometers, where improved optical design and sensor technology have pushed the limits on imaging depths and speed.
Wide-field, high-resolution retinal imaging is one important application empowered by ultrafast SD-OCT. Several retinal diseases may show structural changes in the central foveal region relatively late in disease progression. A wider field of imaging can enable early diagnosis. A fast SD-OCT imaging system can provide the high resolution necessary to resolve the fine structures in the retina over a wide field of imaging.
The research group at the Massachusetts Institute of Technology led by professor James Fujimoto has used a state-of-the-art ultrafast SD-OCT system with the Cobra-S spectrometer to acquire wide-field and high-resolution retinal images (Figure 2).

Figure 2. An ultrafast SD-OCT image of human retina provides both wide-field and high-resolution images. This image was acquired using a Cobra-S OCT spectrometer. No averaging of the data was performed. The image was acquired at a 128-kHz A-line rate, with an expected axial resolution of 3 μm in tissue. Courtesy of James Fujimoto, MIT.
“In ophthalmology, SD-OCT has the advantage that it achieves higher resolution than SS-OCT. Advances in line scan camera and spectrometer technologies have more than doubled imaging speed, while also reducing sensitivity roll off,” said Fujimoto, who led the invention of OCT in 1991 with a seminal paper published in Science3. “The high speeds achieved by state-of-the-art line scan cameras and SD-OCT will be especially important for volumetric imaging applications and en face OCT, where each pixel in the en face image is generated from an axial scan in the volumetric data set.”
OCT has the ability to measure phase of light that is sensitive to small changes in the structure. However, phase imaging requires very high speeds to avoid any corruption of the signal with unnecessary perturbation. A combination of high speed and high resolution is promising a new set of such applications.
One of them is a variant of SD-OCT called traction-force optical coherence microscopy (TF-OCM). Traction-force microscopy (TFM) is a family of techniques that quantify cellular forces via imaging of the deformations the forces induce in the surrounding substrate. Cellular forces are known to play a key role in many processes, including differentiation, development, migration and metastasis, which are critical for studying cancer biology. TF-OCM4 provides a method for measuring dynamic cellular traction forces in highly scattering biological environments with high temporal resolution and high imaging resolution, offering an advantage over traditional 3D-TFM methods. Both the high-resolution and high-speed requirements are aptly met by new ultrafast SD-OCT.

Figure 3. Cell-induced deformations measured with optical coherence microscopy (OCM). False color image generated via automated 3D segmentation of OCM images (a). Red, green and blue channels depict embedded polystyrene beads, contractile NIH 3T3 fibroblast and all remaining scattering signals, respectively. Scale bar = 25 µm. Digital rendering of the 3D displacement field surrounding the contractile cell (b). Scale bar = 50 µm. Courtesy of Steven Adie, Cornell University.
At Cornell University, a TF-OCM system was developed by the research group led by assistant professor Steven Adie. The data from it is used to produce a 3D displacement field map produced from traction forces exerted by the cultured cells (Figure 3). Adie refers to the importance of new spectrometer technology: “Cobra-S provided a noticeable improvement in OCT system sensitivity and roll-off, and we were also able to increase data acquisition speed critical for TF-OCM application.”
The latest developments in SD-OCT are enabling new applications by building on 25 years of continuous innovation in OCT. With interest from top researchers in the field, this technique is set to further the biomedical research and lead to both scientific and commercial breakthroughs.
Meet the author
Nishant Mohan, Ph.D., is vice president of the OCT division at Wasatch Photonics. He has actively participated in strategy, marketing, research and development of OCT for more than a decade. Prior to joining Wasatch, Mohan was part of the R&D team of Bausch & Lomb Inc. in Rochester, N.Y., and a research fellow at Harvard Medical School. He also serves as judge for the annual Prism Awards and is part of the public policy standing committee of SPIE; email: [email protected].
References
1. M.A. Windsor et al. (2018). Estimating public and patient savings from basic research: a study of optical coherence tomography in managing antiangiogenic therapy. Am J Ophthalmol, Vol. 185, pp. 115-122.
2. G.D. Glinos et al. (2017). Optical coherence tomography for assessment of epithelialization in a human ex vivo wound model. Wound Repair Regen. doi:10.1111/wrr.12600.
3. D. Huang et al. (1991). Optical coherence tomography. Science, Vol. 254, Issue 5035, pp. 1178-1181.
4. J.A. Mulligan et al. (2017). Measurement of dynamic cell-induced 3D displacement fields in vitro for traction force optical coherence microscopy. Biomed Opt Express, Vol. 8, Issue 2, pp. 1152-1171.
Comparing Modalities
The table demonstrates strengths and limitations of two main OCT modalities and the impact of the latest developments in technology on trade-offs for users and system developers. Swept-source OCT (SS-OCT) is traditionally useful for applications that require high speeds, such as wide-field retinal imaging. It is also the technique of choice when deeper imaging is required, as in the case of cardiovascular and dermatological applications. The latest developments in spectral-domain OCT (SD-OCT) have made it suitable for high-speed applications. For instance, it can provide wide-field retinal imaging, but without loss in axial resolution of the image. The design improvements have increased the capability of SD-OCT to provide deeper imaging, but SS-OCT is still the technique of choice where very long-range imaging is required, as in intravascular imaging applications. The latest developments in SD-OCT haven’t increased cost or complexity of the systems.
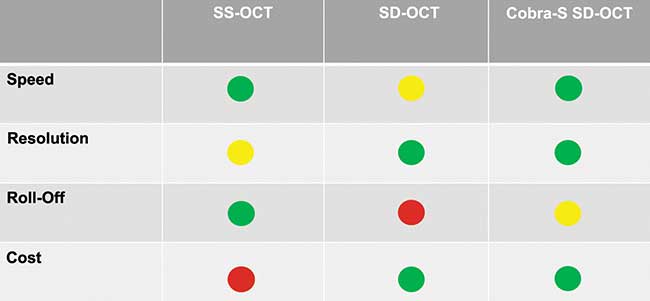
The green circles refer to the strength of the OCT technology, the yellow circles correspond to
acceptable performance, and the red circles refer to relative weakness. These characterizations are meant to indicate a trend, and thorough analysis of specific systems should be made for utilization in different applications.