Attention and investment could revolutionize applications in visioning and treatment of eye-related diseases.
HAO F. ZHANG, NORTHWESTERN UNIVERSITY, AND KIEREN PATEL, OPTICENT HEALTH
First reported in 1991 by Huang and colleagues1, OCT has become an important imaging tool for ophthalmology following innovations over the past three decades. OCT noninvasively provides 3D in vivo optical biopsy with microscopic resolution in the eye at a depth range up to the choroid2. Besides 3D anatomical imaging, OCT can also provide functional information. The most notable functional extension is OCT angiography (OCTA), which can map the microvasculature in the eye without contrast agent and is being investigated in clinical ophthalmology.
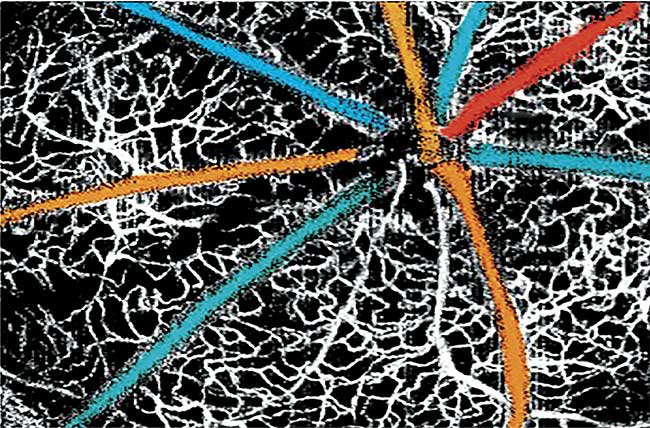
A multicolored retinal blood oxygen saturation map overlaid on a VIS-OCT angiogram. Adapted from Reference 5.
OCTA opens up a new window on better managing a wide variety of blinding diseases through noninvasively visualizing the complete vascular network, including the multilayer inner retinal capillary network and deep choroidal vessels, without using extrinsic labels. More importantly, OCTA is compatible with most existing OCT systems and can be implemented through a software upgrade.
All commercial OCT systems, to date, use NIR light as a result of various economical, clinical, and technical considerations. However, as broadband visible light sources generating low-coherence light from 400 to 2000 nm or more have become available, visible-light OCT (VIS-OCT) device development has gained momentum, as evidenced by the dramatically increasing number of scientific publications and presentations (Figure 1a). There are now many research groups from institutions around the world — such as the Medical University of Vienna; the University of Amsterdam; Shanghai Jiao Tong University; the University of California, Davis; and Oregon Health & Science University (OHSU) — devoted to various aspects of VIS-OCT developments, from optical engineering to signal processing and clinical tests. One startup company, Opticent Health, has also started to commercialize VIS-OCT for both preclinical and clinical usages.
Figure 1a shows the total number of scientific publications from 2013, when VIS-OCT was first reported by the Zhang Lab for retinal oximetry in rodents3, to the end of 2018. Figure 1b shows the annual research funds awarded by the National Institutes of Health (NIH) to conduct various investigations using VIS-OCT. In 2019 alone, over $5 million has been awarded for VIS-OCT research, and NIH investment has steadily increased since 2014. It is clear that VIS-OCT has the potential to become mainstream. One of the original OCT inventors, OHSU professor David Huang, recently commented that VIS-OCT is “a new frontier” for OCT research4.
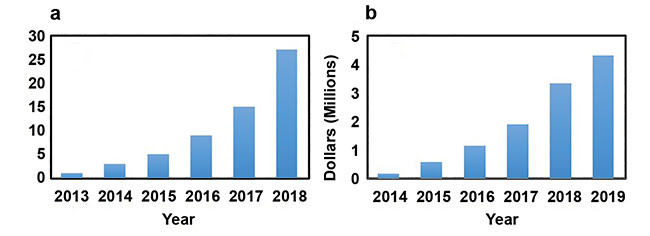
Figure 1. Number of scientific publications on VIS-OCT. Data obtained from Web of Science
and Google Scholar (a). Annual NIH research support for VIS-OCT awarded by the end of 2018.
Data obtained from NIH RePORT (b).
Imaging in mouse, human eyes
The motivations for VIS-OCT development came primarily from the demand for higher spatial resolution and new imaging contrasts to improve disease management at ever finer tissue levels and beyond anatomical alterations. With comparable bandwidth, visible-light illumination significantly improves the OCT axial resolution over and above NIR light. In addition, VIS-OCT can retrieve unique scattering and absorption contrasts from the ocular tissue within the visible spectral range to extract new functional information, such as retinal hemoglobin oxygen saturation3.
Table 1 summarizes the key parameters of the VIS-OCT system developed by Opticent Health. Using a bandwidth of 100 nm, ultrahigh axial resolution can be achieved, which well exceeds the state-of-the-art NIR OCTs. The reported illumination power of VIS-OCT on human studies is 220 µW or less, which is considered eye-safe according to ANSI Z136.1 (2014).
Table 1. Key Parameters of the Latest VIS-OCT System
Figure 2a shows a typical VIS-OCT amplitude fundus image of a wild-type mouse retina. The radial pattern of major blood vessels and the capillary bed are clearly visualized in the fundus image as a result of the strong optical absorption of visible light by hemoglobin. When taking advantage of the motion contrast of the blood, VIS-OCT angiogram (VIS-OCTA) can be achieved in the same manner as the modern OCTA. The angiographic image from the same volume as Figure 2a is shown in Figure 2b. All three layers of the capillaries are clearly visualized at the single capillary level. Figure 2c shows a high-definition B-scan image from a mouse eye, where all of the microanatomic layers are clearly imaged with ultrahigh axial resolution.
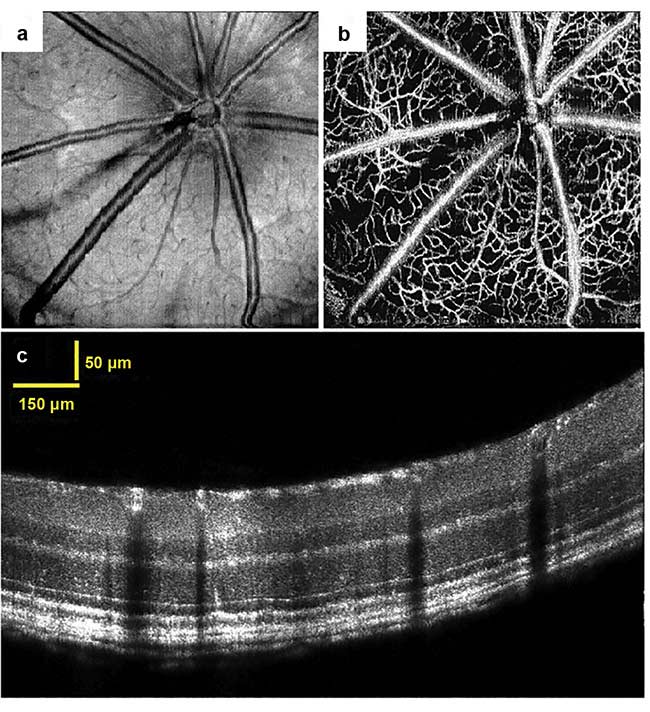
Figure 2. VIS-OCT retinal imaging in mouse. En face fundus image (a). Microangiogram by VIS-OCT (b). A high-definition B-scan image showing all the fine details of the retinal layers (c). Reprinted and adapted from Reference 5.
Researchers from UC Davis and Northwestern University have applied VIS-OCT to image human eyes to measure retinal hemoglobin oxygen saturation and ultrafine inner and outer retinal anatomical sublayers (sidebar). Figure 3 shows a high-definition B-scan image from a healthy human eye. Figure 3a shows the image from the fovea, and Figure 3b shows the magnified view of the area highlighted by a red square. All five outer retinal layers — including the inner/outer segment junction (IS/OS), the cone outer segment tips (COST), the rod outer segment tips (ROST), the retinal pigment epithelium (RPE), and Bruch’s membrane (BM) — are clearly resolved. These minute outer retinal anatomical layers are so far not easily resolvable by NIR-OCTs without being enhanced by adaptive optics.
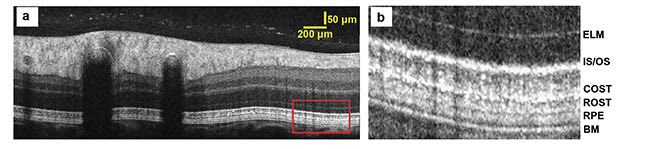
Figure 3. VIS-OCT B-scan image of human retina. ELM: external limiting membrane. Courtesy of Ian Rubinoff/Northwestern University.
Clinical and preclinical uses
Besides ultrahigh axial resolution, another key advantage of VIS-OCT is its sensitivity to new tissue scattering and absorption properties in the visible spectral range. These added sensitivities enable VIS-OCT to explore new applications for clinical management of several blinding diseases.
In the first example, several groups have applied VIS-OCT to quantitatively measure retinal blood oxygen saturation (sO2). It is believed that better understanding of retinal oxygen metabolism could greatly facilitate the diagnosis6 and anti-VEGF (antivascular endothelial growth factor therapy) treatments of diabetic retinopathy and macular degeneration7,8. Figure 4a shows the process of spectroscopic analysis of VIS-OCT signals to calculate sO2. For each 1D interferogram, researchers divide the full spectrum into several subbands (Figure 4a, top). Then VIS-OCT reconstructs B-scan images in each subband to form the basis of spectroscopic analysis. The bottom of Figure 4a shows the B-scan images of a retinal vessel from different subbands using short-time Fourier transform. These spectral signatures can be correlated with optical absorption of both oxygenated and deoxygenated hemoglobin to estimate sO2. As shown in Figure 4b, sO2 in each individual major retinal blood vessel can be extracted. We measured the mean sO2 values in arteries and veins at 95 ± 3% and 72 ± 7% in a mouse, respectively5.
A second example is VIS-OCT’s capability to potentially diagnose different types of glaucoma far earlier — for example, through spectroscopic sensing of neuron damages — than clinically
detectable biomarkers currently can. Figure 4c shows the en face image of the retinal nerve fiber layer (RNFL) and ganglion cell layer (GCL), where retinal ganglion cell axon fiber structures are clearly visualized as a result of the much-improved contrast in the visible-light spectral range. Using a similar short-time Fourier transform-based spectroscopic analysis of the RNFL
(Figure 4d), VIS-OCT can provide insight into ultrastructural damage well before RNFL thinning occurs9.
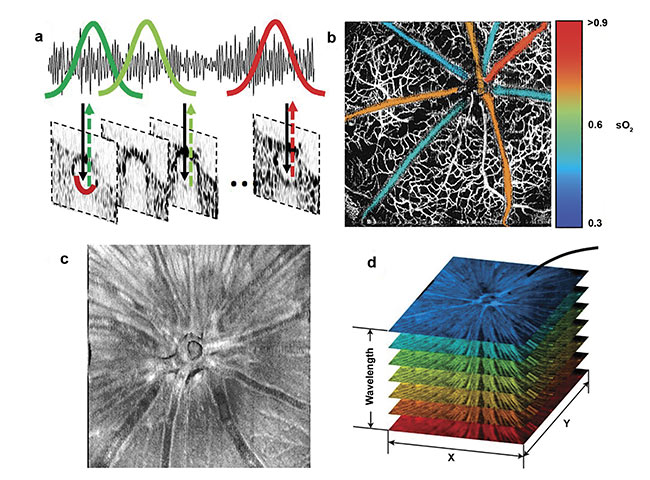
Figure 4. Method to extract sO2 from single retinal vessels (a). Pseudo-colored sO2 map overlaid on VIS-OCT angiogram (b). En face image of segmented RNFL/GCL (c). Spectroscopic VIS-OCT image stack (d). Images (a) and (c) adapted from Reference 3; (b) adapted from Reference 5; (d) adapted from Reference 9.
VIS-OCT represents one of the most exciting new imaging directions for clinical ophthalmology. With superior resolution and the ability to generate accurate functional information, VIS-OCT is likely to change diagnostic and prognostic treatment protocols for a variety of blinding diseases. More OCT research groups and physician scientists are needed to explore its full potential. In addition, the cost of VIS-OCT systems needs to come down to be comparable to existing commercial OCTs and to facilitate broad clinical adoption. Reducing the price of VIS-OCT is predominately constrained by the high cost of its light source; thus, new light sources are needed through continuous technology innovations.
Meet the authors
Hao F. Zhang, Ph.D., is professor of biomedical engineering and ophthalmology at Northwestern University. His lab focuses on new technologies of optical coherence tomography and single-molecule imaging, as well the application of these technologies to biology and medicine. He received his doctoral degree in biomedical engineering from Texas A&M University and postdoctoral training at Washington University in St. Louis.
Kieren Patel (Ph.D., MBA, J.D.) is CEO of Opticent Health, which seeks to commercialize visible-light optical coherence tomography for both clinical and preclinical markets. He co-founded Opticent Health with professor Hao F. Zhang in 2015 in Evanston, Ill. Patel received his doctorate in molecular biology from the University of California, Berkeley and his MBA and J.D. from Northwestern University.
References
1. D. Huang et al. (1991). Optical coherence tomography. Science, Vol. 254, Issue 1178.
2. W. Drexler and J.G. Fujimoto (2015). Optical Coherence Tomography: Technology and Applications. N.Y.: Springer.
3. J. Yi et al. (2013). Visible-light optical coherence tomography for retinal oximetry. Opt Lett, Vol. 38, pp. 1796-1798.
4. S. Binder (Jan. 2019). Ophthalmic imaging modalities: a lot of change in 2 years. EyeWorld, www.eyeworld.org/ophthalmic-imaging-modalities-lot-change-2-years.
5. S. Chen et al. (2015). Measuring oxygen saturation in retinal and choroidal circulations in rats using visible light optical coherence tomography angiography. Biomed Opt Exp, Vol. 6, pp. 2840-2853.
6. R.A. Linsenmeier and H.F. Zhang (2017). Retinal oxygen: from animals to humans. Prog Retinal Eye Res, Vol. 58, pp. 115-151.
7. P.L. Nesper et al. (2017). OCT angiography and visible-light OCT in diabetic retinopathy. Vision Res, Vol. 139, pp. 191-203.
8. W. Liu et al. (2017). Increased inner retinal oxygen metabolism precedes microvascular alterations in rodent model with Type 1 diabetes. Invest Ophthalmol Vis Sci, Vol. 58, pp. 981-989.
9. J. Yi et al. (2016). Optical detection of early damages in retinal ganglion cells in a mouse model of partial optic nerve crush injury. Invest Ophthalmol Vis Sci, Vol. 57, pp. 5665-5671.
Seeing Potential in Retinal Imaging
In 2015, the Zhang group from Northwestern University and Dr. Amani Fawzi, a Northwestern ophthalmologist, reported the first demonstration of human retinal imaging using VIS-OCT. The authors showed that VIS-OCT provides much-improved imaging contrast and axial resolution in visualizing fine anatomical features — of retinal nerve fiber layers and lamina cribrosa, for example — as compared with modern clinical OCTs.
In 2017, the same Northwestern team reported the measurement of retinal oxygen saturation in humans. In that work, the authors imaged healthy volunteers and showed that oxygenation levels decrease with arterial vessel bifurcations after applying a statistical denoising algorithm. In the same year, the Srinivasan group from the University of California, Davis successfully measured retinal oxygen saturation in humans, together with blood flow and hematocrit. Both the Northwestern and UC Davis teams developed spectroscopic analyses to extract blood oxygenation from the full-band VIS-OCT image. Prior to these two reports, only fundus photography-based methods were reported for retinal oximetry, where lack of axial resolution leads to challenging repeatability. Retinal oximetry is believed to be greatly beneficial in managing diabetic retinopathy, macular degeneration, and glaucoma.
Recently, the Northwestern and UC Davis teams started to use the quality of VIS-OCT retinal imaging and VIS-OCT’s ultrahigh axial resolution for clinical management of glaucoma, for example. The UC Davis team corrected the chromatic aberration of VIS-OCT illumination and further manipulated the spectral profiles of VIS-OCT probing light to achieve nearly submicron axial resolution. The Northwestern team developed a speckle reduction method through spatial beam modulation and demonstrated significantly improved imaging quality in resolving minute anatomical features in both the inner and outer retinas. In addition, the Yi group from Boston University further integrated NIR light OCT with VIS-OCT through an achromatizing lens for dual-band retinal imaging, aiming to detect spectral signatures of the retinal nerve fiber layer under various stages of glaucoma.
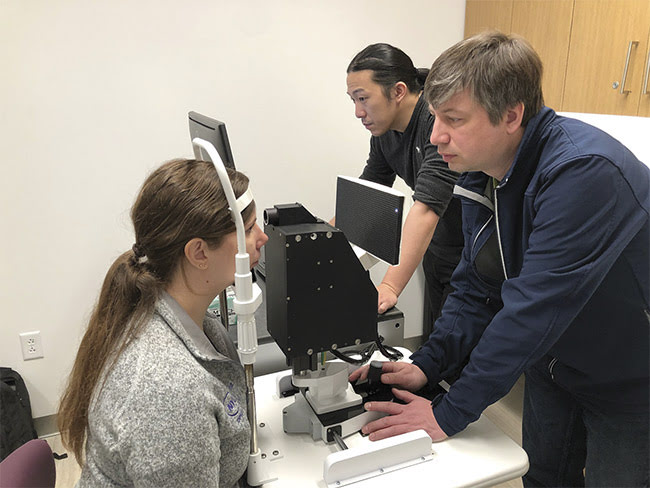
Figure 5. Treatment image. Courtesy of Opticent Health and Joel Schuman/Langone Medical Center, NYU School of Medicine.