Ultrashort-Pulse Lasers Leave Their Mark
THORSTEN FERBACH, COHERENT INC.
Lasers have long been important in manufacturing for their ability to leave a permanent mark for use in tracking and traceability or as a design element. Increasingly, manufacturers are turning to ultrashort-pulse (USP) lasers in the NIR, visible, and UV for high-value marking of materials such as plastics used in automotive design or metal nanostructures in medical
devices.
Permanent marks can be made on a wide range of substrates by different types of material transformation. But virtually all of the different marking applications and processes can be grouped into five basic categories: engraving, photothermal, foaming, photochemical, and black marking.
Here we see one of the next-generation ultrashort-pulse (USP) lasers (Coherent Rapid NX) as a subsystem with integrated optics in a turnkey tool. Courtesy of Coherent Inc.
Engraving refers to ablating material from the surface. The resultant relief pattern can be the final mark, or engraving can be used with laminates to expose an underlying layer that has a contrasting appearance. Here, the same laser can sometimes be used to perform marking and cutting. And occasionally, the marks are filled with some type of compound to enhance the contrast. Subsurface ablation is also sometimes used to create marks as voids inside a transparent material such as glass or sapphire.
Photothermal marking refers to changing the color by localized heating, which causes some type of oxidation or charring. It is widely used to mark low-value organics such as paper and wood by charring, for example, in applications where mark resolution is not particularly demanding. Food cartons and packaging are prime examples.
Foaming is commonly used to produce permanent light marks on dark pigmented plastics. Courtesy of Coherent Inc.
Foaming is used with plastic substrates, where the material is momentarily brought to a boiling point. The resulting foam then immediately hardens as the laser moves to the next location. For black plastic substrate impregnated with carbon, the hardened foam has a lighter color. This is often used with keyboards where die printing cannot withstand repeated finger contact. Because the
level of substrate deformation can be manipulated, foaming can also be used to create tactile marks.
Photochemical marking involves changing the color of the material by using laser light to break chemical bonds. The most common photochemical transformation is reduction: to bleach a colored material and leave a pale mark. But some materials are designed to produce other types of color change. Examples of photochemical marks included master circuit breakers (made of PA 6.6 nylon) and disposable polyamide catheters for medical applications.
Laser marking sometimes relies on photobleaching to produce light marks on a darker background. Courtesy of Coherent Inc.
Black marking refers to the production of black subsurface marks on metal substrates by nanopatterning the metal. The two principal examples are black logo marks on anodized aluminum cases for tablet computers, and identification marks on stainless steel medical devices that can withstand repeated autoclaving without fading.
Different wavelengths needed
Because marking may involve any of these processes and may be used for a wide range of different materials, several different laser wavelengths are used to satisfy all the disparate applications. The 1064-nm NIR wavelength services the broadest range of high-quality marking applications based on photothermal and foaming processes. That’s because this wavelength is a great match for marking metals and many plastics. Years ago, these NIR applications were the exclusive domain of Q-switched solid-state lasers, originally lamp-pumped and then diode-pumped. But today, the market is split between DPSS (diode-pumped solid-state) and fiber lasers, where DPSS lasers tend to be favored where mark quality and resolution are critical considerations, and fiber lasers are usually preferred where cost is the preeminent factor. Both laser types are thus required to properly service this huge market segment.
Frequency-doubled DPSS lasers at 532 nm fill an important niche in creating marks in tougher materials that cannot be efficiently marked at 1064 nm. Examples include metals such as gold and certain glass products. In some semiconductor marking applications, 532 nm is also used where the material bandgap is such that the semiconductor is semitransparent at 1064 nm.
Frequency-tripled DPSS UV lasers at 355 nm are used for photochemical applications. A standout example is nylon, a heat-resistant plastic that is used in master circuit breakers in residential wiring setups. UV lasers also overlap and compete with 532-nm lasers in some application areas. Where speed is critical, 532 nm is preferred, because these lasers are available at higher power. In cases where high resolution is important, 355-nm lasers are desired because they can be focused to a smaller spot size.
Carbon dioxide (CO2) lasers with FIR outputs between 9.4 and 10.6 µm are also used, but for low-value, low-resolution photothermal marks on paper and other organics, because the long wavelength cannot be focused to a small spot.
Thermally based techniques such as engraving and foaming involve processing only very small amounts of material. Yet lasers used in marking applications have average output powers, ranging from a few watts to tens and even hundreds of watts, as overall throughput is directly dependent on average power, and different applications need different throughput speeds.
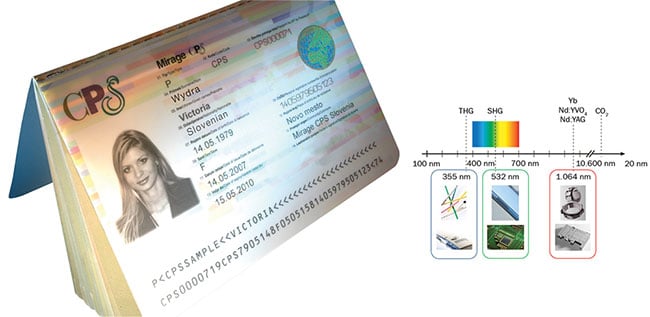
Top right: Spectrum of wavelengths and common marking applications. The majority of marking applications are performed at 1064 nm, but green (532 nm) and UV (355 nm) DPSS lasers service several important applications that cannot be performed at 1064 nm. THG: third-harmonic generation; SHG: second-harmonic generation. Courtesy of Coherent Inc.
Top left: Careful control of the laser foaming process allows it to be used to create tactile marks. Courtesy of Coherent Inc.
Below: Laser engraving is sometimes used with layered substrates, including multipart forms. Courtesy of Coherent Inc.
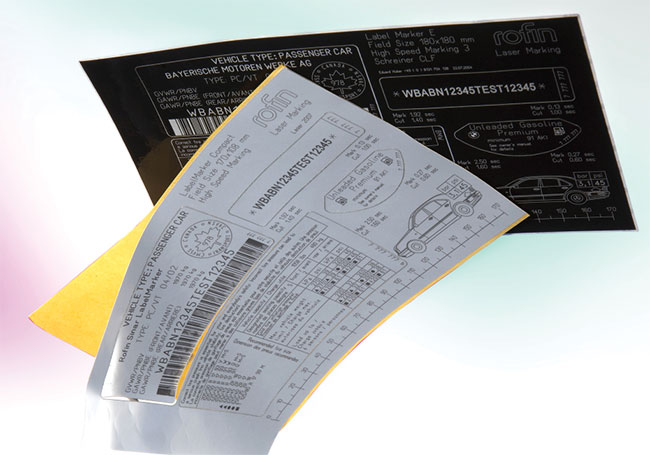
Moreover, marking is virtually always an ancillary process in any production environment, so it is critically important that marking throughput matches the speed of the production line. There is, therefore, an optimum power for each application; if the power is too low, the marking will be too slow to match the production line. But if the power is too high, then the customer is wasting power. And with lasers, higher power generally translates into higher cost, so the marking cost is unnecessarily high.
USP lasers
One of the key trends in laser marking is the increasing use of USP lasers in processes that cannot be optimally performed with nanosecond lasers. A typical example is plastic coated with chromium, as used in some automotive design features. Here, patterning the chromium via ablation with nanosecond lasers can cause thermal distortion of the plastic substrate. In contrast, the higher peak power of ultrashort — picosecond, for example — laser pulses can enable entirely new processes. This so-called black marking entails transforming the metal nanostructure and is quite different from black marks caused by oxidation and charring.
Concurrently, a new generation of industrial picosecond lasers provides the lower capital cost and long-term reliability required to make USP laser marking economically competitive in a much wider spectrum of applications.
An example of black marking involves its use with stainless steel medical devices to meet a new mandate for unique device identifier (UDI) marking of medical equipment. For reusable, nonimplantable devices, the critical date was Sept. 24, 2018. Since that date, Class II devices have had to bear a UDI permanent marking. This regulation encompasses one-time and multiuse stainless steel tools and devices, where "reprocessing" typically refers to autoclaving. This has created a need to produce high-contrast marks in stainless steel that can be discerned by automated readers and that don’t compromise the surface passivation or fade during repeated autoclaving.
For example, using a picosecond laser with an average power of 7 W, a pulse width of <15 picoseconds, and a maximum pulse repetition rate of 1 MHz creates a mark on 1.4301 stainless steel. While these marks appear similar to the black marks produced using nanosecond lasers, microscopic investigation reveals that their actual structure is quite different. The black appearance of nanosecond laser marks on steel primarily arises from the creation of a black oxide. With picosecond laser marking, a major contributor to the high-contrast black appearance is light trapping caused by nanopatterning the metal surface, without significant change in the material composition. Because these are subsurface marks, there is no roughness to trap contamination. The high contrast remains even after multiple autoclaving cycles. Because the surface passivation is not compromised, the black marks do not act as sources of corrosion during this autoclaving. This new marking process is thus ideal for applying UDIs to stainless steel medical devices.
Integrated systems with optional peripherals
Laser and laser system manufacturers can supply a standalone laser, a laser subsystem (rail) consisting of a laser, and a processing (scanning, focusing) head attached to the laser chassis, or a completely integrated marking machine (tool). With the exception of USP laser developments, lasers and laser rails are in a state of maturity and changes are incremental. Rather, it is with integrated tools that technology is changing most in terms of hardware options and sophisticated software peripherals.
In terms of optional hardware, the processing head often includes a vision system, autofocus hardware, or some other type of process monitoring technology. The parts are supported on a work table inside the tool. Although Z axis motion is mandatory, several additional axes can be considered, again, driven by the specific application. Typically, these include X, Y, and often a rotary axis to mark cylindrical parts circumferentially. For convenient and efficient loading and unloading, a common option is to offer a two-station dial table — two identical tables that enable marking on one table while loading or unloading parts outside the closed tool space.
Some examples of black marks created on 1.4301 stainless steel using a Coherent Rapid NX picosecond laser incorporated in a PowerLine laser rail. Courtesy of Coherent Inc.
Importantly, these marking tools make use of standard industrial interface technology to link the different hardware elements that encompass laser operation, various motion axes, and vision systems. In this way, all the software functions are integrated and use a single control interface. Moreover, many industrial production lines now use some type of manufacturing execution system (MES) to monitor, optimize, and control all aspects of product flow.
At its most sophisticated, with the latest integrated marking tools, the laser is increasingly buried within the system, with many users buying a process solution rather than a laser system.
Laser marking is one of the largest market segments and is characterized by incredible diversity in terms of application specifics and the tools needed to support these applications at various points of entry and integration. USP lasers will continue to drive innovations that address emerging issues in marking of high-value substrates, such as stainless steel, in medical and other exacting applications.
Meet the author
Thorsten Ferbach is a business development manager at Coherent for the automotive and automotive supply industry, including applications in marking and materials processing. He joined Rofin (now part of Coherent) in 2007 and holds a degree in electrical engineering.
/Buyers_Guide/Coherent_Inc/c2706
Published: September 2018
Glossary
- laser marking
- Laser marking is a process in which a laser beam is used to mark or engrave a surface by altering its properties or appearance. This technique is widely used for labeling, identification, and decoration of various materials, including metals, plastics, ceramics, glass, and more. Laser marking provides a permanent and high-precision method for adding information or graphics to a wide range of objects.
Key features of laser marking include:
Contactless process: Laser marking is a non-contact...
- photochemical
- The term photochemical pertains to chemical processes or reactions that are initiated or influenced by the absorption of light. Photochemical reactions involve the interaction of light, often in the form of ultraviolet or visible radiation, with molecules, leading to changes in their chemical structure or properties. These reactions are distinct from thermal or non-light-induced chemical reactions.
Key characteristics of photochemical reactions include:
Light absorption: Photochemical...
laser markingultrashort-pulse lasersengravingphotothermalfoamingphotochemicalblack marking CoherentFeatures