The key to successful commercialization of ultrafast technology is developing robust, cost-effective systems with simple push-button operation.
Ultrafast optics is currently a scientifically dominated area with a lot of dynamics in both research and development. Often, complex setups make it challenging to create compact devices that can be employed for applications outside of the laboratory or in medical environments.
But stepping toward commercial approaches goes hand in hand with the development of industry-proven fiber lasers, as well as multifunctional and multimodal microscopy technologies.
Doctoral students adjust the pump laser of a high-repetition rate optical parametric chirped pulse amplifier (OPCPA) at the Munich-Centre for Advanced Photonics (MAP). Courtesy of Thorsten Naeser/Max Planck Institute for Quantum Optics.
Today, ultrashort laser pulses with durations in the range 30 to 50 fs can be generated routinely, corresponding to around 10 cycles of the electric field for visible light. And with novel pulse compression schemes, such as using hollow core fibers, even “single cycle” pulses in the 3- to 5-fs range can be achieved.
Because of the high intensity of the light that can be reached for these tiny fractions of a second, short pulses can drive highly nonlinear processes. In research, these range from straightforward multiphoton microscopic imaging using low-energy pulses, typically over 100 fs, to cutting-edge phenomena driven by amplified pulses greater than 5 fs such as coherent x-ray generation, which is still in its infancy.

Doctoral students adjust a setup for the generation of attosecond light pulses at the Max Planck Institute of Quantum Optics. Courtesy of Thorsten Naeser/Max Planck Institute for Quantum Optics.
Even ophthalmologic and some materials processing applications now use femtosecond lasers, albeit at longer pulse widths of a few hundred femtoseconds. According to Marco Arrigoni, director of marketing at Coherent Inc. in Santa Clara, Calif., until recently the optics that support these applications — ranging from dispersion compensation cavity mirrors to pulse compression prisms to broadband beam delivery optics and so on — were considered mature.
“These applications are not currently limited by the performance of the optics, and the available optics have delivered good reliability and lifetime characteristics when correctly manufactured and used,” he said. “However, the applications space is changing and so must the optics and lasers that support these methods.”
Among the most dynamic areas are optogenetics and three-photon imaging. For these methods, researchers are pushing for longer wavelengths (and higher powers) beyond 1 µm, which has required new lasers with optical coatings (both intra- and extra-cavity) re-optimized to fully exploit the potential of these longer wavelengths.
Specifically, while Titanium:Sapphire lasers with around 250-nm tunable output were a critical advance for enabling multiphoton imaging 10 years ago, longer excitation wavelengths were required for deeper and damage-free multiphoton imaging in live animals. As a result, optics and coating designers eventually developed cavity and beam delivery optics where a single set of optics could cover the entire titanium gain bandwidth from 680 to 1080 nm. In the last couple of years, ytterbium fiber lasers with an integrated optical parametric oscillator (OPO) have been developed as a complementary tunable laser technology to provide higher power at 1 to 1.3 µm, primarily for neuroscience.

Components in an optical setup for the generation of few-cycle laser pulses. Courtesy of Thorsten Naeser/Max Planck Institute for Quantum Optics.
Welcoming these recent developments in fiber laser technology are scientists that include professor Matthias Kling at the Laboratory for Attosecond Physics at Ludwig-Maximilians-Universität Munich and the Max Planck Institute of Quantum Optics in Garching, Germany. He is working closely with colleagues at the Munich-Centre for Advanced Photonics (MAP) and the Centre for Advanced Laser Applications (CALA) to use ultrafast pulses to develop a platform for cancer cell molecular analysis that enables cancer targeting on the level of a single cell.

Matthias Kling (left), a professor at Ludwig-Maximilians-Universität Munich and the Max Planck Institute of Quantum Optics, discusses a setup for the generation of few-cycle laser pulses with one of his senior researchers. Courtesy of Thorsten Naeser/Max Planck Institute for Quantum Optics.
“We see that related setups are becoming significantly smaller and reach already shoe-box sizes, where they have occupied large optical tables in the past,” Kling said. “Pulses with durations of just a few cycles (a few femtosecond pulses) are highly beneficial in terms of reduced cell damage and provide the required increased contrast for label-free imaging.”
Off-the-shelf pulse widths in the 100-fs range are provided by commercial one-box products such as the Chameleon Discovery from Coherent, which offers over 600 nm of tunable output (from 680 to 1300 nm).
“One of the biggest challenges in moving to this longer wavelength platform was the design and fabrication of the optics and multilayer optical coatings in both the OPO and the group velocity dispersion [GVD] precompensator,” said Darryl McCoy, Coherent’s director of product marketing.
Specifically, these had to be carefully optimized to provide dispersion management, to deliver this over an unprecedented wavelength range, and to avoid high internal electric fields that could otherwise lead to coating damage because of the high intracavity peak powers.
“Success in meeting all three criteria with these coatings was critical in reaching our goal of providing both short pulses and the widest tuning range in a turnkey platform supporting broad uses,” McCoy said. “The end result is a new regime in ultrafast laser performance and reliability to match the needs of both research and commercial applications.”
The longer wavelengths emitted from ytterbium fiber-based lasers is supporting some of the latest research in three-photon excitation, particularly in the field of neuroscience. For the same fluorophores used in two-photon excitation, this represents a 50 percent increase in laser wavelength, which is allowing researchers to conduct experiments that monitor activity deeper into the brains of subject animals.
“The main depth-limiting parameter is light scatter — brain tissue is nonhomogenous [and] scatter has a strong inverse dependence on laser wavelength,” McCoy said. “In particular, imaging beyond the white matter in the mouse is difficult, although the rewards are high, as the hippocampus below is a valuable source of information for learning and memory.”
Looking to the future, Kling believes that combining such ultrafast optics technology with nanoscale devices holds great promise for further decreasing the dimensions and required power for certain applications.
“Already now, it is possible to fabricate optical fibers with diameters in the micrometer range that fit into the smallest injection needles,” he said. “Such developments could herald a new age of micro-optical diagnostics and surgery.”
Unlocking ultrafast processes
Ultrafast optics enables scientists to investigate processes that are faster than a picosecond (10−12 s). In this regime, molecular and atomic processes are revealed with unprecedented detail. And in the nascent field of attosecond science enabled by femtosecond laser amplifiers, even electronic processes can be interrogated in real time.
First demonstrated in 2001 by Reinhard Kienberger, Ph.D., along with professor Ferenc Krausz and his group, then at the Vienna University of Technology in Austria, attosecond laser pulses (1 attosecond = 10-18 seconds) are now being generated in many laboratories around the globe, with the latest achievements enabling movies of the motion of electrons to be recorded.
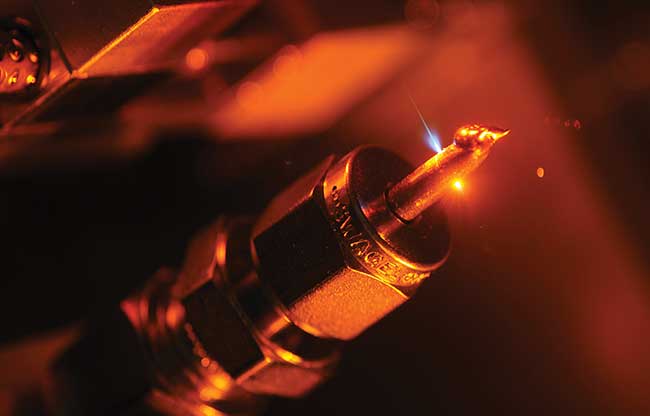
Gas-filled capillary used for the generation of attosecond light pulses in an attosecond beamline (AS-5). Courtesy of Thorsten Naeser/Max Planck Institute for Quantum Optics.
“From my point of view, measuring electron transport along biomolecular chains is the most thrilling application of ultrafast optics recently,” Kienberger said. “During the last years, scientists have been able to generate subcycle laser pulses (carrying only one optical cycle of the electric field) with defined waveforms — a great achievement together with several new measurement techniques that allow insight into ever faster processes and, more important than that, allow [us] to control processes on the atomic timescale.”
Such ultrashort light pulses generated across a wide spectrum of frequencies with arbitrary electric field waveform means that the force the light exerts on electrons in atoms and molecules can be controlled for the first time.
“They have permitted steering bound and free electrons and controlling the directionality of chemical transformations, which might one day lead to novel ways to synthesize drugs,” Ludwig-Maximilian’s Kling said. “It is likely that pulses with tailored and well-defined field waveform will also find other applications in biomedicine.”
“Despite these clearly impressive developments,” he added, “we are still at the beginning of the age of ultrafast optics in biomedical and life sciences, in particular with regard to their applications outside of the laboratory.”
At Menlo Systems GmbH in Martinsried, Germany, Mose Choi, Ph.D, head of International Sales of Fiber Lasers, explains that the biggest challenges at the doorstep of commercialization, regardless of the application, are cost-effectiveness and the lack of widely used end-consumer applications.
“The technology enables nearly limitless possibilities, but it yet has to prove itself against state-of-the-art technologies in both feasibility and pricing,” he said. “Future applications are widely spread among quantum computing, multimodal microscopy, high-speed communications, as well as security applications.”
Clean and Pristine: Ultrafast Cavity Optics
Naturally, high-performance optics require much technical expertise including coating designs that yield multilayer coatings with high reflectivity and low group velocity dispersion, and that avoid high electric fields at layer interfaces, where high-power pulses could otherwise cause damage. But Coherent Inc.’s Marco Arrigoni points out that care of the optics, while it may sound boring and low-tech, is an equally critical consideration.
“One of the areas that we have pioneered at Coherent has been the industrialization of ultrafast lasers including those used in life sciences, where lasers now routinely provide thousands of hours of hands-free performance,” he said. “The laser optics have played a big part in this.”
Cleaning the optics is a crucial step in ultrafast laser maintenance. If ultrafast cavity optics are not maintained or cleaned, Arrigoni noted that laser performance deteriorates. And high peak power of the femtosecond pulses could permanently damage those “dirty” optical coatings.
“For this reason, today we use only materials and assembly/testing methods, and sealed cavities, that ensure the optics inside the laser can’t get dirty — dust, vapors, etc. — in the first place,” he said. “The optics are then just high-reliability internal components that the end user never even has to think about.”