Aluminum oxynitride and magnesium aluminate spinel are mechanically strong, hard and scratch resistant; they are also chemically durable. Both exhibit broadband transmittance from near-UV to mid-IR.
Mohan Ramisetty, Lee Goldman, Nagendra Nag, Sreeram Balasubramanian and Suri Sastri, Surmet Corp.
Single-crystal sapphire has been the material of choice for defense and other applications that require extremely durable optics, but aluminum oxynitride (ALON) and magnesium aluminate (spinel) have emerged for use when those applications need optics in large sizes or with complex geometries.
Table 1 lists the key physical properties of each material. Both have cubic spinel crystal structures that make them transparent in their polycrystalline form, which means they can be produced using conventional ceramic powder processing techniques. Powder processing allows them to be produced in large sizes, complex geometries and large volumes that otherwise would be very difficult or impossible.
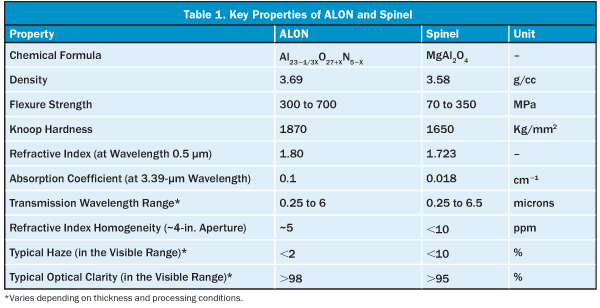
While their crystal structures are similar, some of their mechanical and optical properties are different. ALON’s high hardness, modulus and strength are especially suited for transparent armor; spinel’s extended transmission in the mid-infrared wavelength region (Figure 1) makes it applicable for many IR optics uses.

Figure 1. Transmittance spectra of ALON, spinel and single-crystal sapphire at 2-mm thickness (without antireflection coatings).
How they are made
The manufacturing processes for ALON and spinel are similar to the processes used for any other ceramic materials. However, because these materials need to be transparent, purity and process control are quite a bit more challenging. Between the two, the ALON process is much more robust, has higher yields and produces parts of better quality compared to spinel.
Unlike single-crystal sapphire, versatile ceramic green-forming techniques allow ALON and spinel components to be formed into near-net shapes prior to heat treatment, saving energy and significantly reducing machining costs. The processing steps (Figure 2) for both materials involve:
(1) Powder synthesis.
(2) Green body formation (shaping).
(3) Heat treatment.
(4) Optical fabrication.
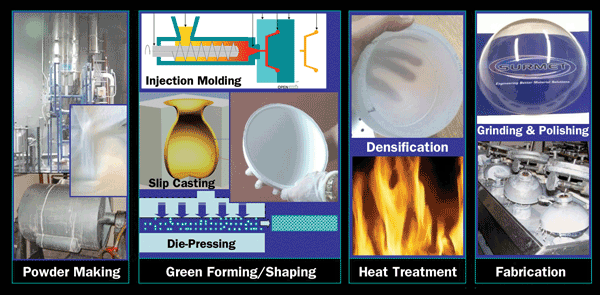
Figure 2. Typical process steps involved in manufacturing ALON and spinel transparent ceramics.
Green bodies can be created by one of a number of forming methods, including cold isostatic pressing, die pressing, injection molding and slip casting. Green bodies have the consistency of chalk and are only partially dense. They are then subjected to a number of heat treatment steps which bring them to full optical transparency. Commercially available ALON components are made using sinter/hot isostatic pressing (HIP) processes. They are virtually inclusion-free and generally known for their high optical clarity, low haze, being tint-free and – most importantly for many critical applications such as reconnaissance and sensor windows – for good refractive-index homogeneity over large areas. Spinel can also be produced using the same basic heat treatment approach.
Another production method for spinel components uses a uniaxial hot pressing followed by hot isostatic pressing (HP/HIP) approach. In this process, a liquid-phase sintering aid such as LiF is generally needed. Unfortunately, many studies have shown that the LiF-based approach leads to serious grain-boundary weakness issues, resulting in low strength and also problems in attaining a high level of refractive-index homogeneity.
Complex geometries, multifunctional features
Optical ceramic components with complex 3-D geometries are required for many applications, including IR military optics. Hemispherical and hyper-hemispherical domes, tangent ogive domes, complex lenses and curved windows are some of the complex geometries possible with powder-based ALON and spinel optical ceramics (Figure 3). Applications that use such components include tri-mode (laser, IR and radio frequency) seeker systems, IR countermeasure systems and aircraft-targeting pod systems.
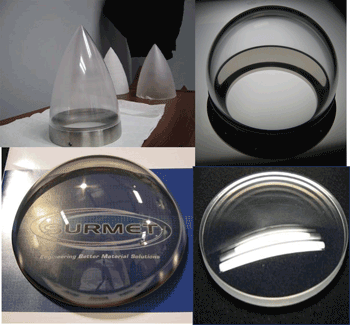
Figure 3. Examples of ALON and spinel components with complex geometries.
Manufacturing components with such complex geometries from single-crystal sapphire is uneconomical because of the costs involved in growing boules and subsequent machining of these shapes from the boules. Like sapphire, ALON and spinel ceramics still need grinding and polishing, but their near-net shape formability in the green stage eliminates huge machining/optical fabrication costs.
Embedded internal architecture within ALON optical ceramic components offers the potential for additional functionality. Some examples of multifunctional components are (Figure 4):
• Domes or windows with embedded grids or meshes for EMI (electromagnetic interference) shielding.
• Domes or windows with embedded antennas.
• Domes or windows with built-in recesses and internal electrical connections for sensors.
Additionally, compliant and integrated internal structures can be tailored to enhance mechanical properties such as toughness, structural integrity or ballistic performance of the component.
ALON-based GRIN optics
Although polymer and glass-based gradient index (GRIN) optics have been
around for quite some time, ALON-based GRIN optics are relatively new
and currently under development in a DARPA-funded research program at
Surmet. GRIN-based lenses have a refractive-index profile that varies
gradually within the material in a controlled fashion. In comparison to
regular lenses, which depend upon curvatures machined into their
surfaces to bend light, GRIN optics can also use controlled gradients
within the material itself to bend the light. This can be used with or
without surface curvature to produce lenses.
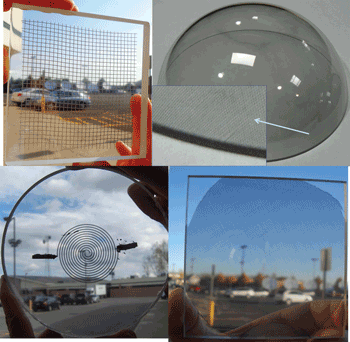
Figure 4. Examples of ALON components with embedded internal architectures.
This additional control can lead to substantial miniaturization and weight reduction in optical systems, as well as reduction in optical aberrations caused by spherical lenses.
When the refractive index varies through the thickness of a lens, it is called axial gradient. Typical applications of such lenses include photographic objectives, because of their significantly low chromatic aberration compared to aspheric lenses. The index profile can also be varied radially (Figure 5), and this type of lens offers both aberration correction and the ability to change the focal length of the lens. ALON-based GRIN optics can be fabricated with significantly large refractive-index gradients (?n), ranging up to 0.04 in both axial and radial profiles. This is achievable in ALON either by changing the composition and/or by adding suitable dopants.
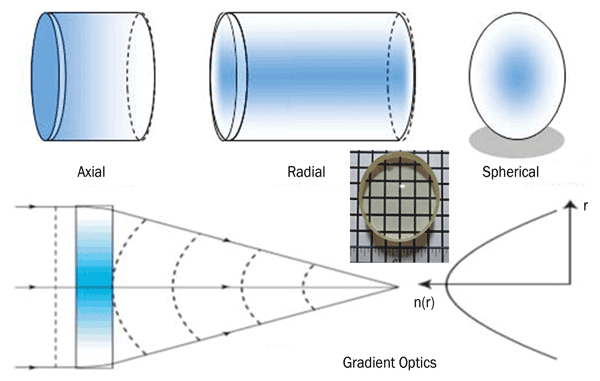
Figure 5. Graphical illustration of GRIN profiles (an ALON GRIN sample is shown in the inset).
Unlike many glasses and polymers, which are only transparent in visible wavelengths, ALON GRIN lenses are transparent in the visible- through mid-IR range. Furthermore, they offer exceptional durability in terms of scratch resistance, strength and high temperature resistance over glasses and polymers. This opens up many other potential applications for ALON GRIN lenses, such as image systems for laser rangefinders, night-vision goggles and unmanned aerial vehicles.
Acknowledgments
The authors would like to acknowledge support from Mark Smith, Uday Kashalikar and Santosh Jha.
References
1. Mohan Ramisetty et al (2013). Transparent polycrystalline cubic spinels protect and defend. American Ceramic Society Bulletin, Vol. 92, No. 2, pp. 20-24.
2. Daniel C. Harris (1998). Durable 3-5µm transmitting infrared window materials. Infrared Physics and Technology, Vol. 39, pp. 185-201.
3. Nagendra Nag et al (2013). Multi-functional windows, Proceedings of SPIE, Vol. 8708, Window and Dome Technologies and Materials XIII, 87080B (June 4).
4. Lee M. Goldman et al (2011). ALON optical ceramic transparencies for window, dome and transparent armor applications. Proceedings of SPIE, Vol. 8016.
5. D.T. Moore (1995). Ch 9: Gradient Index Optics, Handbook of Optics, Vol. 2.