As the technology matures, scientists fine-tune performance.
Dr. Adolf Giesen and Jochen Speiser, University of Stuttgart, and Rigo Peters, Christian Kränkel and
Dr. Klaus Petermann, University of Hamburg
The thin-disk laser is a diode-pumped solid-state laser that produces high output power with both high efficiency and excellent beam quality. Since the first demonstration of the principle, by Adolf Giesen at the University of Stuttgart in 1993,1 the continuous-wave output power extracted from a single disk has increased to more than 5 kW.
In recent years, researchers have concentrated on finding new materials for thin-disk lasers. In many applications, the well-investigated ytterbium-doped yttrium aluminum garnet (Yb:YAG) is still the material of choice, but other applications call for a new material. This is especially true for applications such as tunable lasers or the generation of very short pulses that require a broad gain profile because the limits of Yb:YAG are reached very quickly.
Research has been directed at improving the efficiency of thin-disk lasers, enhancing their wavelength-tuning range and generating high-power short pulses.
Thin-disk design
The thin-disk design employs gain media that have a very short axial dimension of several hundred microns, but a much greater transverse dimension of several centimeters (Figure 1). The disks are antireflection-coated for the pumping and lasing wavelengths at the front side and coated for high-reflectivity at both wavelengths on the back. The crystal is mounted on a heat sink and cooled from behind, so that the thermal gradient is nearly perfectly orthogonal to the surface. Thus, thermal lensing is minimal.

Figure 1. For efficient absorption, the pump light is reflected through the thin disk multiple times.
But because only a small amount of pump light is absorbed by the thin, face-pumped disk, the pump light must be bounced back and forth through the disk numerous times. In practice, a parabolic mirror and deflecting prisms guide as many as 32 passes through the disk. A simple laser resonator can be formed between the highly reflecting back surface of the disk and an output-coupling mirror, or a more sophisticated resonator can employ additional optics to enhance spatial overlap between the pump light and the intracavity mode.
Yb:YAG is the most widely used material for thin-disk lasers because of its long radiative lifetime (~950 μs), its high thermal conductivity (~6 W/m K for typical doping concentrations) and its good mechanical properties. Slope efficiencies up to 70 percent and optical-to-optical efficiencies up to 65 percent can readily be achieved. The highest output power demonstrated so far from a single disk is 5.3 kW (Figure 2). Today, Yb:YAG is the only material in commercial thin-disk lasers. The most powerful commercial unit on the market is currently the Trumpf model TruDisk 8002, which puts four disks in a single resonator to generate 8 kW with an M2 beam quality of 24.
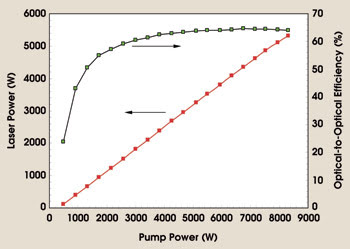
Figure 2. Output power from a single disk can be in excess of 5 kW. Courtesy of Trumpf-Laser.
Straightforward power scaling can be achieved with Yb:YAG thin disks by increasing the size of the pumped area while maintaining the pump power density and other parameters. Thus, the total pump power — and output power — can be increased without significantly changing the average temperature or the thermal gradient. Thus, the good efficiency and high beam quality are maintained (Figure 3).

Figure 3. Increasing the diameter of the pumped area on the thin disk can boost output power while maintaining relatively high efficiency. This plot shows results for several different diameters of 940-nm pump light.
Highly efficient fundamental-transverse-mode operation of the thin disk also is possible: In the lab, we demonstrated 225-W fundamental mode with Yb:YAG, and about 100 W are commercially available from ELS Elektronik Laser System GmbH in Gross-Bieberau, Germany.
Higher power and efficiency
Despite these excellent results achieved with Yb:YAG, there are reasons to consider alternative materials. Higher pump power densities would allow a more compact resonator design without diminishing the beam quality. Even thinner disks would reduce the thermally induced phase distortion, boosting the efficiency of fundamental-mode operation.
A simple way to accommodate both higher pump power and thinner disks would be to increase the ytterbium-doping concentration. Unfortunately, there is a poorly understood quenching process in Yb:YAG that increases with the doping concentration. In practice, this quenching process limits ytterbium concentrations in YAG to 15 percent or lower.
New materials could improve the performance of thin-disk lasers in several ways:
• Materials with a higher thermal conductivity would reduce the temperature rise or allow for higher pump power densities.
• Materials with higher absorption cross sections would allow reduced crystal thickness at the same density of Yb3+ ions.
• Broader zero phonon line absorption would decrease the demands on the pump diodes for direct pumping into the upper laser level, which would reduce the heat generation and the temperature rise or allow for higher pump power densities.
• Materials that show reduced quenching effects compared with Yb:YAG would allow for higher doping concentrations and thinner crystals.
A material with one or more of these desirable characteristics also must fulfill several practical requirements. It should be easy to grow with high optical quality and high purity and be easy to polish to a thickness of 100 μm or less with good optical quality surfaces. The resulting thin disk should have high mechanical strength to allow cleaning, coating and other handling.

Figure 4. This chart shows a comparison of unpolarized absorption cross sections and thermal conductivity at suitable Yb concentration for some promising Yb-doped crystalline materials.
Many host materials can be doped with ytterbium. In Figure 4, some of them are compared with regard to thermal conductivity and absorption cross sections. One promising class of host materials includes the monoclinic double tungstates KY(WO4)2 (known by the acronym KYW) and KGd(WO4)2 (whose acronym is KGW). These materials provide strong and broad absorption bands — an order of magnitude greater than Yb:YAG — enabling efficient pumping at 981 nm into the upper laser level with a small quantum defect. Unfortunately, the benefits of these crystals’ reduced heat generation and thinner disks are at least partially undermined by their poor thermal conductivity.
Another disadvantage of these double tungstates is that they cannot be grown by the standard Czochralski method because of an incongruent melting behavior. Nevertheless, high-optical-quality, single-crystal boules of more than 100 cm3 can be grown by the flux method and are commercially available. Other disadvantages are the strong tendency for cleaving, limiting the production of very thin disks, and the strong anisotropy in thermal expansion, limiting the usability for high-power applications.
Nevertheless, with Yb:KYW we obtained an output power of 72 W under 120-W pumping, resulting in an optical-to-optical efficiency of 60 percent. With Yb:KGW, we demonstrated a slightly lower optical efficiency of 50 percent, with 59 W of output power at 1030 nm.2
Other promising materials for efficient high-power operation are the sesquioxides, such as yttria (Y2O3), scandia (Sc2O3) and lutetia (Lu2O3). Both the absorption cross sections and thermomechanical properties of these ytterbium-doped crystals are superior to those of Yb:YAG.
The sesquioxides’ broad absorption peak around 940 nm is suitable for laser diode pumping. Alternatively, to reduce the quantum defect and the heat load during laser operation, the narrower zero-phonon-line absorption band around 975 nm can be used for pumping.
However, because of their extremely high melting temperatures — about 2500 °C — Czochralski growth is nearly impossible. For that reason, such alternative growth methods as the heat-exchanger must be employed. Nevertheless, the resulting growth of sesquioxides is difficult. The resulting low optical quality has, until recently, imposed severe limitations on the power available from lasers based on these crystals. The best results with Yb:Sc2O3, for example, have yielded an optical efficiency of about 50 percent, with an output of 125 W. Power from Yb:Lu2O3 has been even more limited, approximately 22 W at 42 percent optical efficiency.
Very recently, improvements in crystal growth techniques achieved by Günter Huber’s group at the University of Hamburg in Germany overcame the existing difficulties with sesquioxides, which allowed the fabrication of large monocrystalline boules of high optical quality; i.e., high-purity Yb:Lu2O3. When operated in a classical longitudinally pumped laser setup, the crystals delivered a record slope efficiency of 84 percent, giving rise to the hope that the results in thin-disk lasers could be improved considerably, too.
Larger bandwidths
To achieve tunable laser operation across a broad wavelength range, a broad emission bandwidth is necessary. With Yb:YAG, the tuning range is limited to about 56 nm, between 1000 and 1056 nm (Figure 5). Because the tunable range of the infrared fundamental is limited, the ranges of the visible and ultraviolet harmonics are likewise limited. Thin-disk lasers have proved compatible with harmonic generation: Commercially, up to 50 W of second-harmonic (ELS model MonoDisk-515-MP) and more than 1 W of fourth-harmonic are available (ELS model VersaDisk-515 with extension module DuoWave UV). But the limited tuning range of Yb:YAG prevents that laser from producing, for example, 253.7-nm radiation, which is important for the detection of mercury in a magneto-optical trap.
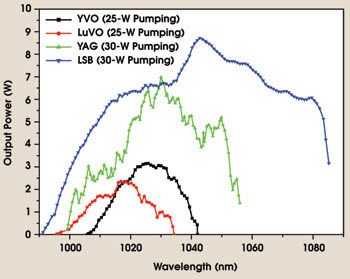
Figure 5. Thin-disk lasers based on various Yb-doped host materials can have very different bandwidths.
Recently, tunable laser operation in a thin-disk laser over a range of nearly 100 nm — between 991 and 1086 nm — was reported for ytterbium-doped lanthanum scandium borate LaSc3(BO3)4, known by the acronym LSB (Figure 5).3 Here the occurrence of three crystal sites for the ytterbium ion with different Stark-splittings results in very broad gain spectra. This laser delivered 40 W of output power at a slope efficiency of up to 56 percent, resulting in an optical-to-optical efficiency of 43 percent.
Many machining applications, such as hole drilling or surface structuring, require ultrashort pulses to produce the desired material modification and high average power for good industrial throughput. The fundamental physics of Fourier transforms tells us that shorter pulses require larger bandwidths. The narrow bandwidth of Yb:YAG prevents it from generating or amplifying sub-picosecond pulses. For that, we must look to other materials.
Yb:KYW is a good alternative: Its broad emission bandwidth is comparable to ytterbium-doped glass and, hence, pulse durations of 240 fs with an average output power of 22 W were obtained in 2002 with this material.4 In a regenerative thin-disk amplifier, we achieved up to 116 μJ with a pulse duration of 250 fs and at a repetition rate of 40 kHz.5 Recently, we increased the pulse energy of this amplifier to 390 μJ at 50 kHz, paying for this with longer pulses.
But as already mentioned, the monoclinic double tungstates are not easy to grow or to handle. Therefore, alternative host materials permitting the generation of pulses in the sub-100-fs regime would be of high interest.
Yb:LSB is a material with a very large tuning range (Figure 5), but it has not been mode-locked yet. The orthovanadates Yb:LuVO4 and Yb:YVO4 are also promising, as they provide 10 times higher absorption cross sections than Yb:YAG and a large gain bandwidth. Thin-disk lasers based on these crystals were demonstrated recently to produce powers exceeding 10 W with slope efficiencies around 50 percent.6
The large bandwidths of these crystals enabled a conventional mode-locked resonator to generate some of the shortest pulses obtained in ytterbium-doped crystalline materials: 58 fs for LuVO4 and 61 for YVO4.7,8 The vanadates also are advantageous in terms of thermal load management because their thermal conductivity when doped with ytterbium is comparable to that of Yb:YAG.
However, the efficient cooling mechanism of the thin-disk lasers allows thinking about the use of materials, which do not have the best possible thermal properties for applications in the midpower range. One example is tetragonal double tungstate NaGd(WO4)2, whose disordered crystal structure ensures that it will have very broad emission and absorption bandwidths. Although this material has the lowest thermal conductivity of all the materials considered suitable for thin-disk lasers, we have generated 16.5 W with a slope efficiency of 51 percent (and an optical efficiency of 40 percent) from a 100-μm-thick disk of Yb:NaGd(WO4)2.
For conventional, high-power thin-disk lasers, Yb:YAG will remain the first choice, but there are still a lot of possibilities for research and innovations with the thin-disk laser concept and new laser materials. This will lead to an advancement of the application of lasers in many fields and could open new applications in the future.
Meet the authors
Adolf Giesen is head of the laser development and laser optics department at the Institut für Strahlwerkzeuge (IFSW) at the University of Stuttgart in Germany; e-mail: [email protected].
Jochen Speiser is a research associate at IFSW; e-mail: [email protected].
Rigo Peters and Christian Kränkel are doctoral students in the group of professor Günter Huber at the Institut für Laser-Physik (ILP) at the University of Hamburg in Germany; e-mail: [email protected] and [email protected].
Klaus Petermann is a staff scientist in the group of professor Huber and is responsible for all activities concerning materials science; e-mail: [email protected].
References
1. A. Giesen et al (May 1994). Scalable concept for diode-pumped high-power solid-state lasers. APPL PHYSICS B, Vol. 58, pp. 365-372.
2. K. Contag et al (2001). Thin disk laser operation and spectroscopic characterization of Yb-doped sesquioxides. Advanced Solid-State Lasers. Trends in Optics and Photonics, Vol. 50.
3. C. Kränkel et al (2007). Continuous-wave high power laser operation and tunability of Yb:LaSc3(BO3)4 in thin-disk configuration. APPL PHYS B, (published online).
4. F. Brunner et al (2002). 240-fs pulses with 22-W average power from a mode-locked thin-disk Yb:KY(WO4)2 laser. OPT LETT, Vol. 27, pp. 1162-1164.
5. M. Larionov et al (2007). Femtosecond thin disk Yb:KYW regenerative amplifier with astigmatism compensation. Advanced Solid-State Photonics, Vancouver, British Columbia, Canada.
6. C. Kränkel et al (2007). High power operation of Yb:LuVO4 and Yb:YVO4 crystals in the thin-disk laser setup. Advanced Solid-State Photonics, Vancouver, British Columbia, Canada.
7. S. Rivier et al (2007). 58 fs pulses from a mode-locked Yb:LuVO4 laser. Advanced Solid-State Photonics, Vancouver, British Columbia, Canada.
8. A.A. Lagatski et al (2005). Yb3+-doped YVO4 crystal for efficient Kerr-lens mode locking in solid-state lasers, OPT LETT, Vol. 30, pp. 3234-3236.