Researchers are seeking to make optical probes that are even safer and smaller.
Michael A. Greenwood, News Editor
Imaging biological systems on the cellular and even molecular level requires a material that is as versatile as it is durable.
Quantum dots possess many of the attributes necessary for this purpose. The strengths of these nanosize semiconductors include their brightness, tunability and stability — the very properties needed when imaging something as complex as the lymphatic system or the spread of cancer or when tracking the movements of an individual cell.
Quantum dots have a few glaring weaknesses, however, that continue to hamper their effectiveness and appeal in many areas of nanobiotechnology research.
Specifically, they generally are made from toxic materials, so the notion of using these tiny yet toxic nanocrystals for in vivo imaging of humans remains unthinkable. The long-term health effects from such exposure are unknown.
And although quantum dots are small, in some cases they are not small enough for the nanoworld. To be useful in certain applications, their core diameter of 4 to 6 nm must be coated with a polymer shell to overcome their incompatibility with water. The result is a bulked-up quantum dot as large as 30 nm in diameter, which is bigger than many of the cellular receptors being targeted for labeling.
Meanwhile, other approaches to biological imaging have a host of issues all their own. Fluorescent dyes, for example, can be plagued by limited brightness and by photobleaching. Simply increasing the concentration of free dyes does not enhance brightness because, above certain concentrations, the dyes “stack” and quench.
To overcome the challenges posed by existing quantum dots and dyes, two research teams are seeking to engineer a new generation of cellular illuminators; during recent experiments, these appeared to offer a smaller size and less toxicity while maintaining the benefits that already existed.
C dots
Although exciting biomedical imaging applications continue to be found for quantum dots, the presence of heavy metals such as lead and cadmium in their core is prompting researchers with professor Ulrich Wiesner’s group in the department of materials science and engineering at Cornell University in Ithaca, N.Y., to look for alternatives.
Their answer to the quantum dot? The C dot.
The team is working with silica-based nanoparticles that have a fluorophore embedded in their core, which seem to exhibit many of the advantageous qualities of quantum dots, including small size, enhanced brightness and high stability, but which are synthesized without heavy metals.
As a result, the C dots — first fabricated at Cornell several years ago — evidently are nontoxic. “This opens up a lot more biomedical and in vivo diagnostics as potential applications,” said researcher Andrew A. Burns.
In the November/December 2007 issue of the Journal of Biomedical Optics, the researchers reported that the material also is an order of magnitude brighter than the constituent dye that they implanted in its core. Additionally, a single C dot can emit 10 or more photons in the time that a single fluorophore can emit one. Their surface can be functionalized to aid targeting or uptake in biological systems, and their size can be customized from tens of nanometers up to microns.
But toxicity remained the biggest concern. To determine how the C dots performed in this respect, the researchers used them in a series of in vivo experiments with mice to gauge whether they have any deleterious effects on living systems.
The C dots were synthesized with Invitrogen Corp. organic dyes (either tetramethylrhodamine isothiocyanate or Oregon Green 488 isothiocyanate) bound within the dots’ core (Figure 1). To this, tetraethoxysilane, a pure silica precursor, was added. The dyed cores were further coated with pure silica to form a core-shell architecture with a final diameter of 30 nm. The probes can be made much smaller, with radii down to 10 nm.
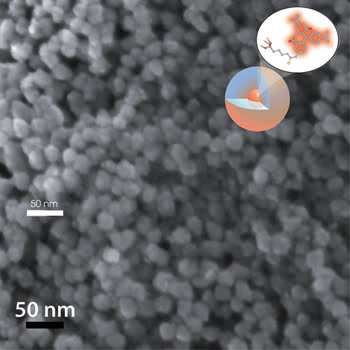
Figure 1. A scanning electron microscope reveals 30-nm-diameter, core-shell fluorescent silica nanoparticles, known as C dots, which contain tetramethylrhodamine isothiocyanate dye, as shown schematically in the inset. Reprinted with permission of the Journal of Biomedical Optics.
The material then was injected into the tail vein of anesthetized mice, at concentrations as high as 0.33 μM, while mice injected with the carrier solution served as a control. The C dots tended to accumulate in the spleen, liver and lungs of the test subjects, but the material became almost undetectable 21 to 60 days after delivery. The mice behaved normally throughout the experiment; at the time of necropsy, no abnormal symptoms were found in any of the assessed tissues or organs.
The researchers said that the silica-based fluorophores exhibited no apparent toxicity in mice and that the experiments indicate that colloidal silica is less toxic than silica in its crystalline form.
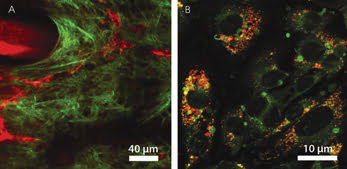
Figure 2. Multiphoton fluorescence microscopy reveals C dots (red) labeling blood vessels in a mouse’s tail after injection. Second-harmonic imaging of collagen (green) shows the surrounding tissue structure. Red autofluorescence from a hair follicle is shown at the upper left (A). On the right are mouse prostate carcinoma cells labeled with C dots (red) functionalized with the cell-penetrating HIV-1 TAT oligopeptide, used to trace cell position in further studies of metastasis (B). The membrane structure is labeled with DiO membrane stain (green). Reprinted with permission of the Journal of Biomedical Optics.
To measure imaging performance, the team tested the particles in several scenarios (Figure 2). In one application, they were tested as tracers for sentinel lymph node mapping. The researchers found that the lymph node was fluorescent 30 s after injection and that it remained visible for up to an hour. In other applications, the C dots were conjugated with peptides to determine how well they could be tuned toward a specific function. These treated particles quickly endocytosed into intracellular vesicles. Untreated particles, meanwhile, exhibited negligible uptake. Lastly, the research team labeled various cells with differently colored C dots. They found that, 5 h after injection, cancer cells retained their green tag and that bone marrow cells could be identified easily by their red fluorescence.
Brightness, however, is a domain in which the material lags behind its rival. The C dots developed so far are not as bright as quantum dots: Compared with quantum dots of similar size and with similar emission wavelengths, they are within a factor of two to three.
Burns said that this is a parameter they want to improve to the extent that C dots’ brightness might even equal that exhibited by quantum dots. “We are working on ways to make them even brighter.” Additionally, as they consider possibly commercializing the material, the researchers are seeking to create C dots that emit at longer wavelengths for improved tissue penetration.
The method used to create the C dots likely can be extended to multiple other types of dyes. Further down the road, the modular architecture of the dots could be exploited to create particles capable of multiple functions. This “lab on a particle” approach could incorporate imaging, drug delivery, sensing and targeting abilities into a single probe.
Slimmer quantum dots
When imaging on the cellular level, every nanometer matters.
An optical probe that is too large can block — or even hinder the function of — the very object that it is attempting to illuminate.
Researchers at MIT in Cambridge and at Oxford University in the UK report that they have devised a surface-modification technique that makes small quantum dots even smaller. This is achieved by applying a suite of recently developed water-soluble ligands, circumventing the need for the bulky coating of polymers that now are used widely to overcome the hydrophobic character of quantum dots.
The result is a quantum dot with more than a few nanometers trimmed off its waist, making it potentially a more effective tool in imaging applications where space is extremely tight, such as in neuronal synapses. The ligand also appears to allow flexibility in how a quantum dot is used (Figure 3), such as in the specific labeling of cells.
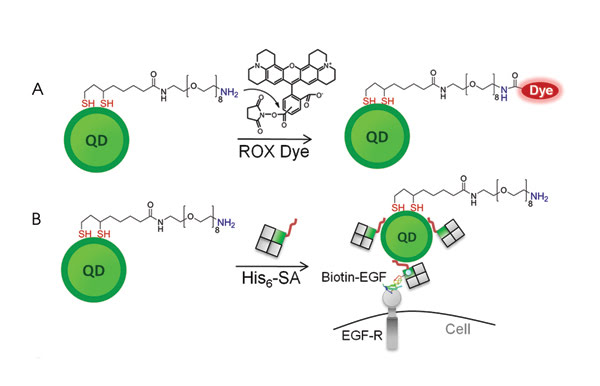
Figure 3. Researchers have functionalized quantum dots for cellular imaging. Bifunctional surface ligands present primary amine groups that can be covalently derivatized; e.g., with a fluorescent dye (A). The quantum dot (QD) surface also is accessible to polyhistidine domains that can be used for metal-affinity-based anchoring of proteins for cell targeting (B). Courtesy of MIT.
Although a number of experiments with surface modifications to these optical probes have been done before, there usually has been a trade-off in terms of performance. For example, ligands such as mercaptoacetic acid markedly reduce the size (down to ∼6 to 8 nm in diameter), but it also causes the probes to bunch together rapidly and contributes to poor performance under acidic conditions.
The researchers overcame these shortcomings by using a group of dihydrolipoic acid-PEG-derived ligands (recently developed at MIT) that terminate in amine or carboxylic acid functional groups. These were exchanged with the hydrophobic ligands now commonly used on quantum dots. They applied these ligands to quantum dots with a CdSe core and a ZnCdS shell and with emission wavelengths ranging from 558 to 605 nm.
“We’ve reduced the size by a factor of about two. We want to make the size as small as possible,” said Wenhao Liu of MIT. Details of the group’s research appeared in the Jan. 30 issue of the Journal of the American Chemical Society.
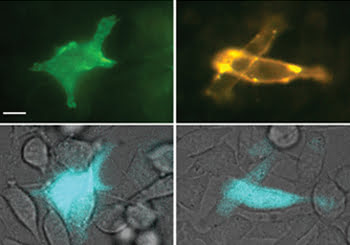
Figure 4. Quantum dots modified with His6-streptavidin are used specifically to probe the interaction of biotinylated endothelial growth factor (EGF) with EGF receptors on transfected HeLa cells (top left). At top right, the quantum dots have been modified further with a red-emitting fluorescent dye, yielding dual-color (red + green = yellow) fluorescence. Bottom panels show corresponding phase contrast images. A yellow fluorescent protein nuclear transfection marker is overlaid in blue. Courtesy of MIT.
The investigators used dynamic light scattering to measure the quantum dots functionalized with the latest ligands and found that they had diameters of ~9.9 and 11.4 nm, respectively; the size of commercial quantum dots currently available ranges from 14 to 60 nm in diameter. They also determined that adding Cd to the shell increased quantum yield of their modified quantum dot by ~40 percent.
The ligand developed by the researchers also appears to contribute to improved dispersion of quantum dots in solutions where fluctuating pH levels are a factor, an area where traditional quantum dots can experience difficulty. The group found that the quantum dots remained stable in terms of brightness after three days of exposure to pH levels ranging between 5.0 and 9.5.
Additionally, the modified quantum dots were tested in several labeling experiments to see whether the ligand would reduce the incidence of nonspecific binding — where the probe tends to latch randomly onto whatever is near and not to what is necessarily being targeted for study. The researchers found that quantum dots capped with the ligand exhibited minimal nonspecific binding.
“It offers a way to do a specific labeling of cell structures,” said MIT researcher Andrew B. Greytak. Potential applications include cell labeling and single-particle imaging.
He said that the group wants to experiment with its surface-modified quantum dots in various biological applications, such as labeling and tracking, to further assess their performance and behavior.