Careful control of fabrication steps is key to superior performance from the ubiquitous ultrafast laser material, titanium:sapphire.
Keith Heikkinen and Mark Anderson, Saint-Gobain Crystals
Ultrafast lasers – with femtosecond or picosecond pulse widths – support a growing range of applications, from precision micromachining to multiphoton imaging to attosecond physics. Titanium:sapphire is the most widely used gain material in ultrafast lasers, but optimizing its crystals presents significant technical obstacles.
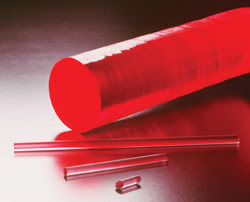
Titanium:sapphire has powered a revolution of turnkey simplicity in ultrafast lasers.
Although Ti:sapphire’s wide gain bandwidth of approximately 650 to 1100 nm makes it ideal for both tunable laser output and mode-locked laser operation in the femtosecond pulse width regime, its potential drawbacks must be overcome.
First, it is a vibronic emission system with a 3.2-ms upper-state lifetime, leading to low gain. From a laser designer’s viewpoint, this low gain necessitates pumping with a high-intensity green laser source and using cavity optics with minimum loss, such as double-chirped broadband cavity mirrors.
For crystal manufacturers, the inherently low gain must be maximized in every way possible. The three most important aspects are the figure of merit equation, the titanium-ion concentration and the orientation of the crystal and lasing axes.
From an OEM vendor’s viewpoint, product uniformity is key.
The figure of merit is defined as the ratio of the absorbance at the pump wavelength – 532 nm – to the reabsorption at the peak emission wavelength of 800 nm. Laser emission is due to Ti3+ ions, but the presence of oxygen in the sapphire matrix causes a small amount of the Ti3+ to be oxidized to Ti4+. This higher valence state is responsible for parasitic reabsorption, so a low Ti4+ concentration is important to a high figure of merit and to higher gain.
Ti:sapphire is grown by slowly drawing a cylindrical boule vertically from a hot liquid melt of titanium and aluminum oxides. Although some manufacturers attempt to use very tight control of the boule drawing conditions to minimize the amount of Ti4+, Saint-Gobain Crystals of Hiram, Ohio, has found it more effective to use a patented annealing process incorporating a hydrogen-rich atmosphere to reduce virtually all of the rogue Ti4+ to Ti3+.
The advantages of this approach are that it allows the boule growth conditions and figure of merit to be independently optimized, provides active control over the figure of merit and leads to uniformity throughout the boule, enabling a large number of highly consistent rods from a single boule for OEMs. Most important, however, is that the method consistently delivers high figure of merit values, typically anywhere in the 300- to 500-nm range.
Increasing total titanium-ion concentration raises gain but also increases lattice defects and makes the lattice physically weaker. At ion concentrations above 0.3 percent, these problems cause yields to drop precipitously, specifically because of cracking caused by thermal gradients and stresses during the boule growing process. Also, pushing to higher ion concentrations results in a vertical concentration gradient, which can significantly lower the useful yield, thus raising the cost while lowering the product quality for longer crystals cut from such a boule.
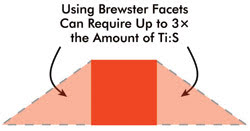
To spread the power in Ti:sapphire amplifiers, the Ti:sapphire must have a large aperture-to-length ratio. Facets are cut at just off normal incidence. Brewster’s angle facets would require wasting a huge amount of material.
Early Ti:sapphire lasers used ion concentrations in the 0.1 to 0.15 percent range. Today the demands of smaller oscillators and ultrashort amplifiers have driven Ti:sapphire fabricators to push the concentration to 0.2 and even 0.25 percent.
Optical axis alignment
Losses are minimized in ultrafast oscillators by cutting the Ti:sapphire crystal with end facets at Brewster’s angle. However, sapphire is a hexagonal crystal favoring lasing action with the electric field vector perpendicular to the C-axis. So it is very important to cut the Brewster windows at the correct orientation. In a worst-case scenario, if the C-axis is aligned parallel to the Brewster window transmission plane, the crystal acts as a self cross-polarizer, preventing lasing.

Because of the low gain of Ti:sapphire, it is vital to create Brewster facets with their transmission plane – e-vector – perpendicular to the C-axis of the Ti:sapphire crystal.
Fortunately, as the boule is drawn from the hot melt, the C-axis is naturally oriented perpendicular to the drawing axis. And because the cylindrical laser rods are cut parallel to this axis to maximize yield, the C-axis is automatically in the correct plane. But this still leaves the issue of rotation of that plane. Saint-Gobain uses x-ray crystallography for this purpose. Specifically, each rod is rigidly mounted in a special jig and placed in an x-ray machine. The diffraction pattern then registers the precise orientation of the C-axis relative to the mounting jig, which is rotated to the correct angle in the Brewster grinding/polishing station. As a result, the tolerance for this relative alignment in the finished laser rod is within 30 min.
Meeting laser trends
Commercial Ti:sapphire lasers fall into two categories: oscillators and amplifiers. Oscillators operate at repetition rates up to 100 MHz, with pulse energies at the nanojoule level. The pulses often are amplified in either a regenerative or multipass amplifier, with repetition rates at the kilohertz level and pulse energies up to the millijoule level. Not surprisingly, oscillators and amplifiers need Ti:sapphire crystals prepared in two distinct ways.
To support the low gain operation in an oscillator and provide superior mode performance, Ti:sapphire rods are supplied as thin cylinders or rhomboids. Losses are minimized by cutting the cylinder ends at Brewster’s angle.
For amplifiers, a larger beam cross section is necessary to physically spread the power and avoid damage to the amplifier components. Commercial amplifiers with crystals as wide as 30 mm are not unusual and typically are supplied with facets cut just off normal incidence, rather than at Brewster’s angle. The reason is that, with a large clear aperture relative to the crystal length, cutting the ends at Brewster’s angle would dramatically increase overall crystal volume and, hence, price. Instead, the facets are coated with an antireflection coating. Manufacturers usually offer a broadband coating to support standard pulse lengths – e.g., up to 200 fs – with wide wavelength tunability, and a coating usually optimized at 800 nm, which supports compressed pulse durations as short as 10 fs.
An exciting new market trend is smaller oscillators with crystals as short as 3 mm and with a clear aperture as small as 1 mm. The oscillators’ lower power makes maximizing gain and minimizing lasing threshold particularly critical. The crystals usually are supplied with a square cross section, simplifying mounting and providing a better surface contact for cooling through the mount.
Enabling diverse applications
Applications for ultrafast lasers have expanded in recent years because of the advent of turnkey, highly integrated Ti:sapphire systems. For example, ultrafast oscillators are now widely used for multiphoton excitation methods in microscopy. They produce diffraction-limited, three-dimensional images, enabling biologists to use markers such as green fluorescent protein and long-wavelength recombinant DNA tools such as m-cherry to study expression of a wide variety of genes. These techniques allow the investigation of key processes such as morphogenesis, the study of how physical feedback controls the expression of regulatory genes, producing embryonic features of the correct spatial characteristics.
The ability to drive multiphoton processes also enables amplified ultrafast systems to perform cold-ablative micromachining with unprecedented precision and 3-D control, including within relatively transparent substrates, such as glass, to create microfluidic tools, and to pattern thin films for the electronics and display industries. Ultrafast lasers also are used to cut the cornea in the “all-laser” version of lasik surgery.
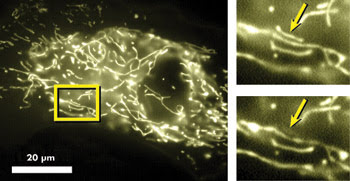
Femtosecond pulses from a Ti:sapphire laser can be used to remove a single mitochondrion with no damage to nearby structures such as other mitochondria. Courtesy of Mazur Group, Harvard University.
In a technique that combines 3-D imaging and machining, life scientists are using ultrafast lasers to perform microsurgery and visualize sequelae; e.g., the deliberate damaging of tiny blood vessels in rat brains to artificially create an ischemic stroke. And at even higher resolution, scientists are using the same technique to destroy individual genes or subcellular organelles in developing cells.
Other applications include generating short-wavelength, or x-ray, pulses, producing and detecting terahertz radiation for imaging applications such as security and screening, for experiments in attosecond physics and for generating highly accelerated subatomic particles. One goal of the latter process is to produce an affordable proton beam that oncologists could use to provide improved spatial selectivity in the radiation treatment of tumors.
Ultrafast applications have grown because laser manufacturers have packaged this sophisticated technology in a way that makes it readily accessible to end users, many of whom are not laser experts. In turn, laser crystal fabricators have supported the growth with products that deliver performance optimized for specific uses, greater reliability and reduced cost.
Meet the authors
Keith Heikkinen is laser materials product manager at Saint-Gobain Crystals; e-mail: mailto:[email protected]. Mark Henderson is optics products manager at Saint-Gobain; e-mail: [email protected].